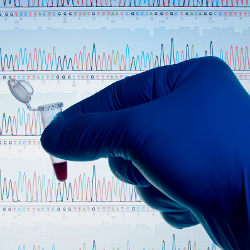
As scientists look to unravel the secrets of life and address a diverse array of challenges involving medicine, agriculture, and more, they increasingly are tossing aside their test tubes and microscopes and diving directly into the genome.
Over the last decade, synthetic biology has emerged as an intriguing, if not remarkable, tool that could fundamentally change the world. The nascent concept, based on creating new sequences of DNA from scratch and rearranging existing ones, already is being used to mimic biological systems, create synthetic biological systems, and actually program biological systems.
It is no small matter. “In the latter half of the 20th century, scientists realized that DNA was the software of living systems and it’s possible to transfer code from one organism to another in order to program cells with new and different functions,” explains Timothy Lu, an associate professor in the Department of Electrical and Computer Science at the Massachusetts Institute of Technology (MIT). However, since the turn of the century, he says, “Researchers have incorporated more complex elements of computing and memory. By assembling genes in a specific way and creating toggles and circuits, it’s possible to produce desired behaviors, and program specific functions into cells.”
Make no mistake, DNA synthesis and sequencing are advancing at a rapid rate. “These technologies are matching or exceeding Moore’s Law,” Lu says. What is more, the ability to program genes will likely change things in profound ways. It could knock out afflictions such as Malaria, cancer, and Huntington’s disease, but also offer a way to steer clear of controversial genetically modified organisms (GMOs) in plants and introduce designer animals and humans. Says Daniel Gibson, vice president of DNA Technologies at Synthetic Genomics, Inc. and associate professor at the J. Craig Venter Institute, a leading genome research center: “As with any new area of science and technology, synthetic genomics has the potential for great societal benefit, but also the potential for harm.”
Beyond the Test Tube
Since the beginning of time, humans have focused on altering the genetic code of plants and animals through selective breeding, hybridization, and other techniques. Modern computing and science have taken the concept to an entirely new level, allowing researchers to achieve results that were previously impossible, or to compress years, decades, and even centuries of gene editing research into weeks or months by rearranging genes and redesigning biological pathways.
Modern synthetic biology is rooted in research from the turn of the current century. At that time, researchers Jim Collins and Tim Gardner of Boston University found a way to create a “toggle switch” with E. coli bacteria; essentially, the engineered cells could hold and maintain a single bit of memory. Around the same time, researchers Michael Elowitz and Stan Leibler of Princeton University built a ring-oscillator that allowed a cell to operate like a mini-clock. “This showed that it was possible to program complex, artificial functions into cells,” Lu points out.
Unlike silicon computing, which relies on ones and zeros to build code into software, biological systems require circuits engineered from DNA, RNA, and proteins. “The DNA interacts with proteins and RNA inside the cell, and the collision between these different parts is what leads to our ability to compute information,” Lu explains. “One challenge with biological systems is that, unlike silicon where you can wire together components and ensure they are isolated from the rest of the world, it’s not easy to do this with biological systems. In addition, they aren’t modular by default; it becomes more of an analog programming task.”
To be sure, programming biology is inherently challenging. “Building circuits inside cells is not a highly scalable approach. The amount of energy that a cell needs to drive different circuits is quite significant,” says Herbert Sauro, an associate professor of bioengineering at the University of Washington. “Unlike electrical systems, there’s a scalability issue and the fact that you need more and more independent proteins to build larger and larger circuits. Evolution did not build large computing systems inside a cell. It had to go beyond the cell and create a multi-cell system, such as the brain, to gain enough computing power Ultimately, every ‘transistor’ in biology has to be unique, so this limits the size of things you can do.”
“Ultimately, every ‘transistor’ in biology has to be unique, so this limits the size of things you can do.”
Yet, knowledge of synthetic biology is advancing at a rapid pace. Better heuristic methods, new design tools, more powerful computers, and increasing big data capabilities are transforming the field. One of the most important breakthroughs came with a gene-editing tool called CRISPR, which “finds the right place in the genome—out of 3 billion places—and cuts it,” says George Church, a professor of genetics at Harvard Medical School. Essentially, the technology allows researchers to expand their toolkit beyond merely adding genes; it lets them delete or change genes to suit their needs. Today, CRISPR is widely used for research on humans, animals, and plants. It is inexpensive, typically costing less than $100 in a lab that is already set up for standard molecular biology, and many government agencies around the world have accepted the technology. “They are saying it doesn’t need to be regulated,” Church adds.
The combination of CRISPR technology, its low cost, and the ability to avoid government regulation has important ramifications. For example, in the agricultural space, CRISPR is “a non-GMO way to do very precise editing,” Church explains. This could reduce the need for lengthy, and sometimes expensive, testing. Genetically designed foods, such as corn, soybeans, and potatoes, could be commercially available within five years. Many of these crops would be easier to grow, more drought-resistant, and more resistant to insects and diseases. They might also grow faster and produce larger yields.
However, the concept goes far beyond field crops. A U.S. company, Recombinetics, is currently using CRISPR and a related technology dubbed TALEN to produce dairy cattle without horns, making them easier to handle. Meanwhile, synthetic biology is now being used to identify spoiled or infected food, and researchers in China have engineered beagles with about double the typical muscle mass, making them suitable for police and military work. Guangzhou General Pharmaceutical Research Institute boasts it produces between 2,000 and 2,500 of these dogs annually.
Yet, much of the work in synthetic biology centers on humans. For instance, Church is working on the complex task of programming pig genomes so pig organs such as livers, kidneys, hearts, and lungs would be compatible with humans, thus expanding the current boundaries of transplantation. He and others are also focusing on developing virus-resistant cells that could be used to eliminate mosquitos or their ability to carry Malaria, which currently kills upward of 650,000 humans per year. “It would target a very small fraction of about 3,500 species of mosquitos,” he explains.
In fact, the possibilities are limited only by the creativity and ingenuity of research teams and further advances in the science, Church says. “The technology could have a profound impact on health and medicine. It’s likely that we will begin to see clinical trials in programmable biology and, after testing gene therapies in animals, move forward to a small set of humans. If it is effective within that group, it will then move onto a larger set of humans.”
DNA by Design
Synthetic biology and genetic re-engineering also introduce ethical and moral questions. Designer dogs many elicit concern, but designer humans fall into an entirely different category. Should parents be allowed to choose characteristics they desire for children—say, blue eyes, or a more muscular physique? Should some parents (essentially those who are more affluent) have access to a technology that allows them to produce babies that are more likely to live longer? What if someone decides to weaponize or sabotage genes in order to introduce disease or cause other problems?
“We should not be dismissive of the potential consequences, including ecological fallout, of the technology,” Church says. “Oftentimes, we think we understand a system and later discover that we really don’t understand it.” Nevertheless, he is optimistic about synthetic biology and its potential impact on the planet. “We slog along and often find we are better off with the technology than without it. With programmable biology, there are opportunities to make life and the world better.”
Adds Lu: “Those who work in the field understand the need to monitor and surveil the technology so that it is not used in nefarious ways. There is now a huge emphasis on ethics and safety.”
One of the biggest questions is how genetic modifications will play out over time in the real world. Although it is theoretically possible for a gene to mutate in an unintended way and introduce dangers and risks, it is far more likely that, as genetically designed genes become part of the natural environment and succumb to evolution, they will eventually fizzle out and lose their characteristics. “These organisms are usually just a little bit ‘sick’ because we are taking up resources that should be used for growth,” Lu explains. “If they can shake off the engineered piece, they’re happier. So, over multiple generations, the beautiful, engineered component may go away; the organism jettisons the engineered parts in order to grow faster.”
Another question is whether scientists can construct more elaborate systems—basically, a new and different type of computer—from biologically engineered components. Sauro points out that all biological systems have reactants bouncing around and colliding with one another, all of which produces “noise.” Evolution exploits this noise, but it is also possible it could be used to compute information. “It’s an open issue about whether there is any advantage to building a computer from biological systems,” Sauro points out.
For now, synthetic biology is marching forward. Over the next couple of decades, Gibson says the technology will usher in huge changes in medicine, biofuel, nutrition, agriculture, and more. “We are taking 4 billion years of evolution and decoding how the biological processes work on a genome. … Right now, we have only a small section of the overall code. In the computing world, we would not have enough to boot up the computer. But in the biological world, we have now built pieces of DNA large enough that we can produce self-replicating cells. This gives us the opportunity to bang on the problem and find tailored applications.”
Vora, S., Tuttle, M., Cheng, J., and Church, G.
Next Stop for the CRISPR Revolution: RNA Guided Epigenetic Regulators, The FEBS Journal, 10.1111/febs.13768. http://onlinelibrary.wiley.com/doi/10.1111/febs.13768/epdf
Chavez, A., Pruitt, B.W., Tuttle, M., Shapiro, R.S., Cecchi, R.J., Winston, J., Turczyk, B.M., Tung, M., Collins, J.J., and Church, G.M.
Precise Cas9 Targeting Enables Genomic Mutation Prevention. http://biorxiv.org/content/biorxiv/early/2016/06/14/058974.full.pdf
Pires, D.P. Cleto, S., Sillankorva, S., Azeredo, J., and Lu, T.K.
Genetically Engineered Phages: A Review of Advances over the Last Decade, Microbiology and Molecular Biology Review, 80:523–543. June 1, 2016. http://mmbr.asm.org/content/80/3/523.short?rss=1
Jusiak, B., Cleto, S., Perez- Piñera, P., and Lu, T.K.
Engineering Synthetic Gene Circuits in Living Cells with CRISPR Technology, Trends in Biotechnology, Volume 34, Issue 7, July 2016, Pages 535–547. http://www.sciencedirect.com/science/article/pii/S0167779915002747
Join the Discussion (0)
Become a Member or Sign In to Post a Comment