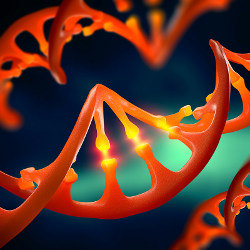
Altering the genetic code of plants and animals is not a job for the faint of heart. Nevertheless, in research labs around the world, scientists are increasingly peering into the cellular structures of living things—and recombining DNA and RNA molecules to produce everything from new tomatoes to new medicines. “The tools and technologies used for viewing and manipulating genetic materials have become more widely available and much easier to use,” observes George Church, a professor of genetics at Harvard Medical School and a pioneer in genomic research.
It is no small matter, even if the matter involved is at the molecular level. CRISPR, a powerful gene-editing toolkit, is advancing the field of programmable biology by leaps and bounds. It allows researchers to reconfigure genes and create new versions of things. Another technology, cryo-electron microscopy (Cryo-EM), is helping scientists peer into genetic material at a resolution that was once unimaginable. They can view the intricate structures of proteins, nucleic acids and other biomolecules, and even study how they move and change as they perform various functions. Both of these tools, as well as more advanced computing models, have introduced a brave new world to genetic research.
“Although these two techniques are very different, they both are reshaping biology and genetic engineering,” states Eva Nogales, a professor in the Department of Molecular and Cell Biology at the University of California, Berkeley, and senior faculty scientist at Lawrence Berkeley National Laboratory. “CRISPR and Cryo-EM allow researchers to perform an array of tasks faster and better.”
Adds Richard Henderson, research scientist at the Medical Research Counsel Laboratory of Molecular Biology in Cambridge, U.K., and a recipient of the 2017 Nobel Prize in Chemistry for his pioneering work on Cryo-EM, “We are at the cusp of remarkable advances in agriculture, medicine, and many other fields. These technologies will reshape science and the world.”
Cracking the Code on CRISPR
In only a few short years, the ability to reengineer the genetic structure of living things has moved from obscure research labs to the mainstream of science. CRISPR, which stands for Clustered Regularly Interspaced Short Palindromic Repeats, beckons with the promise of producing better tomatoes, insect-resistant grains, malaria-resistant mosquitos, and new types of pharmaceutical drugs to combat conditions ranging from sickle cell anemia and Alzheimer’s disease to cancer. Users can perform direct operations on genes by modifying and recombining molecular structures. “As the technology has advanced, the need to build everything from scratch in a lab has been replaced with commercially available products that produce effective results,” Church says.
Indeed, commercial firms with names like Synthego, Inscripta, and Twist Biosciences have developed kits that advance gene editing in much the way same way visual programming replaced the need to manually write endless lines of code for some software application. Although these firms take aim at the task through approaches that range from providing molecular resources to computational tools in software packages, the common denominator for the end-user is an ability to conduct research faster, more effectively, and at a lower cost. In fact, gene-editing tools that once had a price tag extending into the billions of dollars are now available for less than $1,000. Essentially, “Any cell biology lab can use CRISPR,” says Nogales, who visualizes CRISPR molecules using cryo-EM.
For example, Synthego, which Church is affiliated with, has introduced kits designed to address different gene editing tasks. Its $1,495 Gene Knockout Kit (GKO) drops powerful capabilities into the hands of researchers. It taps predictive software and automation tools that help a researcher select a human gene to modify. It then applies a synthetic RNA gene to direct a protein to the specific location required for a DNA cut. The firm claims this toolkit has boosted the accuracy of CRISPR editing methods from around 50% to as much as 80%, or even more. The net result is an ability to cycle through variations of edited genes faster, speeding research and development for new procedures and drugs.
Paul Dabrowski, co-founder and CEO of Redwood City, CA-based Synthego, has said the firm’s gene editing system reduces the time it takes for a scientist to perform gene edits from several months to approximately one month. This, he has noted, helps researchers focus on results and outcomes, rather than the mechanics of an experiment.
“We are at the cusp of remarkable advances in agriculture, medicine, and many other fields. These technologies will reshape … the world.”
Nogales says that while CRISPR tools fundamentally change the nature of research, they also present challenges. For one thing, because of uncertainty about errors caused by systems, CRISPR is not yet been approved for medical use by the U.S. Food and Drug Administration. For another, there is a learning curve associated with the technology. “Making a cut in the wrong place could be very deleterious. This is one of the reasons why CRISPR is used for agriculture more than human treatment, but this will likely change over the coming years.”
Further advances in software and algorithms will drive smarter and better gene editing tools, Nogales adds. For instance, Inscripta, head-quartered in Boulder, CO, has focused on developing a biological genetic engineering framework that resembles the all-in-one capabilities of a personal computer, while San Francisco-based Twist Biosciences is developing a system that places custom strands of synthetic DNA—the As, Ts, Cs, and Gs that serve as building blocks for biology—on semiconductor chips. This allows researchers to make up to a million CRISPR edits with a single chip, rather than using multiple systems and software to accomplish the task. The company’s self-described “smart algorithm” informs users within seconds whether the sequence they are testing can be synthesized.
Cryo-EM Enters the Picture
Although gene editing has introduced powerful capabilities into the research lab, scientists continue to struggle with understanding the mechanical functions of basic biological structures. From the invention of the microscope in the 13th century to more advanced forms of electron microscopy, improving resolution and reducing noise—particularly at extremely high levels of magnification—has proved vexing. “Obtaining clearer images is an ongoing challenge,” states Craig Yoshioka, research assistant professor and co-director of the Pacific Northwest Cryo-EM Center at Oregon Health Sciences University (OHSU) in Portland, OR.
For instance, issue with cryo-electron microscopy is that bombarding a frozen sample with electrons can vaporize the specimen. As a result, Yoshioka says, scientists must essentially collect their images in “low light,” thereby reducing specimen damage, but also resulting in noisy data. The resulting noise makes it more difficult to view the behavior of the molecules and understand how they react to different conditions.
Meanwhile, another technique, called X-ray Crystallography, can produce a three-dimensional (3D) image of a molecular structure at high resolution by measuring how diffracted X-ray beams scatter from crystallized molecules, but is difficult to apply to all samples. “It can be exceedingly difficult to get proteins to crystalize, sometimes nearly impossible,” Yoshioka explains.
Cryo-electron microscopy fundamentally changes the equation. Researchers place biological specimens under a transmission electron microscope and study them under cryogenic temperature conditions: −130°F or less. The system produces digital images that are run through specialized algorithms that dramatically reduce noise and sharpen the image using a method of frame alignment that studies particle behavior in different images. “The software processes the images and identifies the values of the key parameters,” says Henderson, who pioneered imaging techniques that, along with fellow 2017 Nobel Prize winners Jacques Dubochet and Joachim Frank, led to modern Cryo-EM.
Henderson says Cryo-EM addresses a basic problem with conventional electron microscopy: the interaction of electrons with organic matter causes a breakdown in their molecular structure, which generates a high level of visual noise. “It’s a bit like looking for a roe deer in a forest with dappled sunshine. It’s not easy to pick them out because they’re disguised,” he explains. To bypass the problem, Cryo-EM combines more advanced hardware with image-processing software that averages the position and behavior of thousands of individual particles and extrapolates the data to produce much clearer images of a biological structure. As a result, Cryo-EM can achieve atomic-level resolution models of complex, dynamic molecular assemblies.
Conventional electron microscopy is “a bit like looking for a roe deer in a forest with dappled sunshine. It’s not easy to pick them out because they’re disguised.”
Nobel laureate Frank, a professor of biochemistry and molecular biophysics at Columbia University in New York, says advances in graphics processing units (GPUs) and better algorithms have revolutionized the field. “Speed is no longer a problem, with the emergence of GPU software and clever algorithms,” he explains. Moreover, the field is continuing to advance and incorporate new computational methods. For example, “There are now software platforms … that combine different packages under one umbrella and provide interoperability among them so that the user has a choice about which operations to combine for best performance,” he explains.
The result, Yoshioka says, is a new era in understanding the mechanisms of life at a molecular scale. Although Cryo-EM microscopes can cost $6 million or more, their use lets researchers visualize biological molecules at an atomic scale, and see them in their natural state. In contrast, X-ray crystallography requires scientists to order billions of molecules into well-ordered crystals, which can complicate the process of understanding of how they appear or function in a living cell. Using Cryo-EM, “We are better able to understand how proteins behave, how different substances or drugs affect them, and how modifications can change the way a drug binds to the protein,” Frank says.
Beyond Image
Genetic research is also leading biologists down the path of other computational methods that extend the boundaries of programmable biology. For instance, at the University of Groningen in the Netherlands, bio-technologists have used a modeling method to redesign the enzyme aspartase and convert it into a catalyst for asymmetric hydro-amination reactions that produce larger quantities of the substance. Working with researchers in China, the group was able to produce high volumes of extremely pure building blocks of aspartase that could be used in pharmaceuticals and other bioactive compounds.
Meanwhile, at the University of Massachusetts, Amherst, researchers led by computational biophysicist Jianhan Chen are developing sophisticated computer modeling algorithms and molecular simulation models that allow researchers to study a newly recognized class of substances called intrinsically disordered proteins (IDPs). These proteins contain highly flexible 3D structural properties that are extraordinarily difficult to observe. Chen’s technique relies on sheer computational power, because high-resolution imaging techniques like X-ray crystallography and nuclear magnetic resonance (NMR) cannot provide data about the highly flexible and fast-changing nature of these proteins.
Nogales believes these different genetic observation and engineering techniques will continue to break barriers and further advance science. “We are beginning to understand biology, chemistry, and physics at deeper and broader levels than ever before. We are now studying molecules that were almost unknown in the past, and we are putting the knowledge to work through gene editing tools such as CRISPR. We will see enormous changes in the biological world as a result of these techniques.”
Noble, C., Adlam, B., Church, G.M., Esvelt, K.M., and Nowak, M.A.
Current CRISPR Gene Drive Systems are Likely to be Highly Invasive in Wild Populations, eLife, e 2018;7:e33423. DOI: https://doi.org/10.7554/eLife.33423.
Cheng, Y., Glaeser, R.M., and Nogales, E.
How Cryo-EM Became so Hot. Benchmarks. Volume 171, Issue 6, 30 November 2017, Pages 1229–1231. https://doi.org/10.1016/j.cell.2017.11.016.
Wright, VW., Liu, J., Knott, G.J., Doxzen, K.W., Nogales, E., and Doudna, J.A.
Structures of the CRISPR genome integration complex. Science, 20 Jul 2017: eaao0679. DOI: 10.1126/science.aao0679.http://science.sciencemag.org/content/early/2017/07/19/science.aao0679
Russo, C.J. and Henderson, R.
Microscopic Charge Fluctuations Cause Minimal Contrast Loss in Cryo-EM, Ultramicroscopy, Volume 187, April 2018, Pages 56–63. https://doi.org/10.1016/j.ultramic.2018.01.011
Join the Discussion (0)
Become a Member or Sign In to Post a Comment