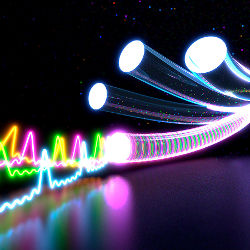
Since optical fibers were first deployed for communications in the 1970s, the number of bits per second a single fiber can carry has grown by the astonishing factor of 10 million, permitting an enormous increase in total data traffic, including cellular phone calls that spend most of their lives as bits traveling in fiber.
The exponential growth resembles Moore’s Law for integrated circuits. Technology journalist Jeff Hecht has proposed calling the fiber version “Keck’s Law” after Corning researcher Donald Keck, whose improvements in glass transparency in the early 1970s helped launch the revolution. The simplicity of these “laws,” however, obscures the repeated waves of innovation that sustain them, and both laws seem to be approaching fundamental limits.
Fiber researchers have some cards to play, though. Moreover, if necessary the industry can install more fibers, similar to the way multiple processors took the pressure off saturating clock rates.
However, the new solutions may not yield the same energy and cost savings that have helped finance the telecommunication explosion.
Optical fiber became practical when researchers learned how to purify materials and fabricate fibers with extraordinary transparency, by embedding a higher refractive-index core to trap the light deep within a much larger cladding. Subsequent improvements reduced losses to their current levels, about 0.2 dB/km for light wavelengths (infrared “colors”) near 1.55 μm. A laser beam that is turned on and off to encode bits can transmit voice or data tens of kilometers before it must be detected and retransmitted. In ensuing years the bit rate increased steadily, driven both by faster transmitters and receivers and by fiber designs that minimized the spread of the pulses.
As the pace of improvements began to slow, researchers realized they could send more information through fiber by combining light of slightly different wavelengths, each carrying its own stream of data. The beams are multiplexed into a single fiber and demultiplexed at the other end using high-tech devices akin to prisms that separate white light into colors.
Adoption of this wavelength-division multiplexing, or WDM, was greatly aided by erbium-doped fiber amplifiers. These devices splice in a moderate length of specialty fiber containing a trace of the rare-earth element, which is pumped with a nearby laser to amplify any passing light within a range of wavelengths. Crucially, this amplification occurs with no need to convert the light to an electrical signal and back again, or even to separate the different colors. Signals can thus travel thousands of kilometers in the form of light.
The widespread adoption of WDM in the 1990s transformed the conception of optical communication from a single modulated beam to a complete spectrum like that familiar for radio waves. The seemingly narrow “C-band” of erbium used in most amplifiers corresponds to a bandwidth of roughly 10 THz, theoretically enough to carry as much as 20 trillion bits (Tb) per second of on/off data. Systems offering scores of wavelength channels were built to take advantage of this previously unheard-of capacity.
A critical—and still open—question is whether systems can become cheaper with SDM than with multiple separate fibers.
Unfortunately, the rapid fiber installation boom was motivated by extraordinary demand projections that proved unrealistic, resulting in a period of excess fiber capacity. Nonetheless, overall traffic has continued to double every two years or less, so after a few years increased capacity was once again needed in high-traffic parts of the network.
To provide this capacity, companies adopted a long-standing research vision of “coherent communication” into the marketplace in about 2010. Rather than representing bits as the presence or absence of light, this technique, widely used in the radio spectrum, encodes data in the phase and the amplitude of the light wave. Although the number of symbols per second is still limited by the available bandwidth, coherent communication allows each symbol to represent multiple bits of information, so the total bit rate increases. Typical systems now transmit 100 Gb/s on each wavelength, or 8 Tb/s over 80 WDM channels, in a single fiber.
Nonlinear Shannon Limit
In theory (the information theory attributed to Claude Shannon at Bell Laboratories in 1948), the number of bits that can be packed into a symbol is limited by the base-2 logarithm of the signal-to-noise ratio. Increasing the power can increase the bit rate, but only gradually.
For optical fibers, however, increased optical power changes the dielectric constant, and thus the propagation, of the optical signal. “There are extra distortions, and some of them you cannot compensate,” said René-Jean Essiambre of Bell Laboratories in Crawford Hill, NJ, which was recently acquired by Nokia (and named Nokia Bell Labs). “These distortions act like noise,” and ultimately nullify any advantage of increased power.
Interestingly, because the nonlinear effects caused by the data on one wavelength channel affect all other channels in the fiber, the net result is a limit on the total number of bits per second in all channels combined. Essiambre and his colleagues have calculated this limit for specific network configurations, and have concluded modern coherent systems are quite close to it.
The limitations on bit rate become especially stringent for very long distances. In addition, realistic reductions in fiber nonlinearity cause only a modest improvement in capacity, Essiambre said. “To increase that number is very difficult because it’s a logarithm.”
To reduce the nonlinearity of conventional fibers, researchers have tried making the core out of pure silica or spreading the light over a larger cross-sectional area, said David Richardson, deputy director of the Optoelectronics Research Centre at University of Southampton in the U.K. “Significant progress has been made,” Richardson said, but “you’re not going to get a factor of 10” reduction in nonlinearity.
In contrast, a 1,000-fold reduction in the nonlinearity has been demonstrated using a fiber that confines the light to an empty core within a periodic “photonic bandgap” material for the cladding. Unfortunately, because of the logarithm and other effects, “the benefits don’t scale linearly,” Richardson said, so “you maybe get a factor of three” improvement in performance. Moreover, the fibers have so far shown an order of magnitude greater loss than conventional fibers, so photonic bandgap fibers are in “the dim and distant future.”
Space-Division Multiplexing
An approach that is “perhaps a little less radical,” space-division multiplexing (SDM), could involve either multiple cores within a single cladding or a fiber that supports several spatial modes rather than just one. Multicore fibers, for example, are “not particularly controversial,” Richardson said, adding that most people accept that the fibers can be operated independently. Even if spatial modes get mixed during their travel, the digital signal processing used in coherent systems can disentangle them as it does for polarization modes and in current application to multiple-antenna radio systems.
A critical—and still open—question is whether systems can become cheaper with SDM than with multiple separate fibers. Researchers have demonstrated simultaneous amplification of different spatial modes by incorporating optical gain into the cladding they all share. “This is where the technology may provide an advantage,” Richardson said, as erbium amplifiers did for WDM.
One company already championing integrated components is Infinera Corp., but Geoff Bennett, the company’s director of Solutions and Technology, is skeptical about SDM. “I’m not going to say never, but for the foreseeable time horizon it’s just not going to happen.”
A major problem is that SDM requires different fibers. “Deploying new fiber is literally the last resort that any operator would consider,” Bennett said, noting recent submarine cable installations have used large-area fibers because their lower nonlinearity is particularly advantageous on those long links.
SDM systems would also require different connectors, splicing, and other infrastructure. “None of that ecosystem that’s been developed over the last 20 years will work” for SDM, Bennett said. Although some links are heavily oversubscribed, “in general there’s plenty of unlit fiber out there” from the boom of the early 2000s. Lighting up a spare fiber from a cable containing scores of them will require a chain of amplifiers every 80 km or so, he admits, but “they’re not that expensive and they never break.”
Lower-Hanging Fruit
Coherent technology has expanded the raw capacity of existing fiber, Bennett said, but there are still opportunities to improve the operational and cost dimensions of network performance. Digital processing was first introduced at receivers, allowing for greater capacity as well as compensation for signal distortions. In what Bennett calls the “second coherent era,” processing is being incorporated at transmitters as well. “That gives you a number of options.”
One such option is the construction of “superchannels,” multiple wavelengths that can be squeezed closer in frequency without interference by shaping the pulses. Tapping the frequency space between neighboring channels “allows you to unlock a lot more capacity in the fiber,” Bennett said; in a typical case, growing from about 8 Tb/s to about 12 Tb/s.
Sean Long, director for Product Management at Huawei, also regards SDM as a question mark for the future, although his company has a “small group” looking at it. “Theoretically, that’s the direction we need to go,” but “there’s a lot of things that we need to develop,” he said. “It’s still too complicated.”
Also, “We still have things we can do before that,” Long said, potentially including erbium amplifiers in the unused spectral region known as L band. “Currently we are more focusing on the spectral efficiency” by exploiting transmission-side digital signal processing. “The flexibility is there already. Now we need to figure out how we can make the best combination for certain applications.”
Energy Crisis
However industry addresses bit-rate limits, other challenges are coming, which were the subject of a May 2015 meeting on “Communications networks beyond the capacity crunch.” Co-organizer Andrew Ellis of Aston University in Birmingham, U.K., had previously analyzed the implications of the nonlinear Shannon limit. Unfortunately, “there are equal problems across the rest of the network,” such as software protocols, he said.
If fiber nonlinearities require the use of duplicate fibers and other components, “it’s difficult to see how you’re going to sustain” the historical reduction in energy cost per bit that has driven network expansion, Ellis said. “Every time we’ve introduced a new generation, there’s been a factor-of-four improvement in performance and the energy cost has only gone up by a factor of two.”
Even if energy reduction continues, the total energy use by communications networks is projected to rival all other energy use within two or three decades, Ellis said. “We are going to use a greater and greater amount of energy if the demand keeps growing.”
Hecht, J. Great
Leaps of Light, IEEE Spectrum, February 2016, p. 28.
Ellis, A.D., Suibhne, N. M., D. Saad, D., and Payne, D.N.
Communication networks beyond the capacity crunch, Philosophical Transactions of The Royal Society A 2016 374 20150191; DOI: 10.1098/rsta.2015.0191. Published 25 January 2016, http://rsta.royalsocietypublishing.org/content/374/2062/20150191
Richardson, D.J.
New optical fibres for high-capacity optical communications, Philosophical Transactions of The Royal Society A, 2016 374 20140441; DOI: 10.1098/rsta.2014.0441. Published 25 January 2016, http://rsta.royalsocietypublishing.org/content/374/2062/20140441
Andrew Ellis
Boosting Bandwidth, Physics World, April 2016, p. 17, http://www.unloc.net/images/news/AndrewEllis_PhysicsWorld_finalarticle.pdf
Join the Discussion (0)
Become a Member or Sign In to Post a Comment