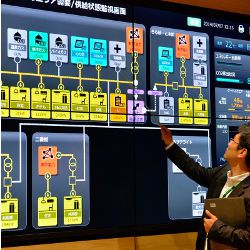
By 2030, the world’s population is projected to be 8.5 billion and increase to 9.7 billion by 2050 and 11.2 billion by 2100. Half of humanity today lives in cities. Many cities are experiencing exponential growth as people move from rural areas in search of better jobs and education. Consequently, cities’ services and infrastructures are being stretched to their limits in terms of scalability, environment, and security as they adapt to support this population growth. Visionaries and planners are thus seeking a sustainable, post-carbon economy20 to improve energy efficiency and minimize carbon-emission levels. Along with cities’ growth, innovative solutions are crucial for improving productivity (increasing operational efficiencies) and reducing management costs.
Key Insights
- Many cities in Asia, Europe, and North America are pursuing smart city projects.
- A smart city is a complex system, meaning even a single vulnerability could affect all citizens’ security.
- Future research must address high energy consumption, security, privacy, lack of investment, smart citizens, and other related challenges to enable secure, robust, scalable smart city development and adoption.
A smart city is an ultra-modern urban area that addresses the needs of businesses, institutions, and especially citizens. Here we should differentiate between a smart city and smart urbanism. The objective of these concepts is the same—the life of citizens. The architects of ancient cities did not take into consideration long-term scalability—housing accessibility, sustainable development, transport systems, and growth—and there is no scalable resource management that may be applied from one decade to another. Unfortunately, smart urbanism is not well represented in smart cities’ development. Smart urbanism must also be considered as an aspect of a smart city, including information-communication technologies. In recent years, a significant increase in global energy consumption and the number of connected devices and other objects has led government and industrial institutions to deploy the smart city concept. Cities’ demographic, economic, social, and environmental conditions are the major reasons for the dramatic increase in pollution, congestion, noise, crime, terrorist attacks, energy production, traffic accidents, and climate change. Cities today are the major contributors to the climate problem. They cover less than 2% of the Earth’s surface yet consume 78% of the world’s energy, producing more than 60% of all CO2 emissions (http://unhabitat.org/).
Innovative solutions are imperative to address cities’ social, economic, and environmental effects. Those solutions involve three key objectives:
Optimized management of energy resources. This objective could be realized through the Internet of Energy (IoE), or smart grid technology. The IoEa,b connects energy grids to the Internet, dispatching units of energy as needed, representing a set of distributed renewable electricity generators linked and managed through the Internet. The IoE enables accurate, realtime monitoring and optimization of power flows;
Decentralized energy production. The IoE concept allows consumers to be energy producers themselves, using renewable energy sources and combined heat and power units; decentralization enables smarter demand-response management of consumers’ energy use; and
Integrated business models and economic models. These models describe how organizations should deliver and reap benefits from their services (such as transport, energy consumption, and charging tolls); such models must be designed to support city development.
Note the “smart city” label is not a marketing slogan. A city is “smart” if it provides better efficiency for urban planning through a variety of technologies. Smart cities are also defined, according to Anthony Townsend in his book Smart Cities (W.W. Norton & Company, 2014), as “places where information technology is combined with infrastructure, architecture, everyday objects, and our bodies to address social, economic, and environmental problems.” The European Parliament proposedc this definition: “A smart city is a city seeking to address public issues via information and communication technology (ICT)-based solutions on the basis of a multi-stakeholder, municipality based partnership.” This is quite broad, encompassing many fields, while the Japanese definition is more specific, focusing on energy, infrastructure, ICT, and lifestyle. From these definitions, we deduce ICT plays a pivotal role in developing a city that can adapt to the needs of its citizens. By leveraging advanced power systems, networking, and communication technologies, a smart city aims to enhance the lives of its citizens and optimize territorial, economic, and environmental resources. Smart cities promise multiple benefits:
Safety and security. This includes surveillance cameras, enhanced emergency-response services, and automated messages for alerting citizens; realtime information about a city should be available;
Environment and transportation. This entails controlled pollution levels, smart street lights, congestion rules, and new public-transport solutions to reduce car use;
Home energy management. Options include timely energy billing, optimal energy management, saving, perhaps, 30%–40% on electricity bills; the European Commission estimates approximately 72% of European electricity consumers will have smart meters by 2020;
Educational facilities. More investment is needed to improve educational opportunities for all, lifelong learning, education through remote learning, and smart devices in classrooms;
Tourism. Preserving a city’s natural resources promotes the growth of tourism; additionally, smart devices offer direct and localized access to information;
Citizens’ health. Using new technologies could improve people’s health; citizens need full access to high-quality, affordable healthcare, and wireless body-area network technology—including sensors attached to the body or clothes and implanted under the skin—can acquire health information (such as heartbeat, blood sugar, and blood pressure) and transmit it in real time or offline through a smartphone to remote servers accessible by healthcare professionals for monitoring or treatment.
Despite this potential, many elements must be understood and considered before cities are able to reap the benefits. Here, we describe some of the basic concepts and architectural components of smart cities, including energy management (ISO 50001), smart homes, vehicular networks, smart grids, and quality of life (ISO 37120). We then examine recent smart city projects around the world, identifying some of the challenges and future research opportunities. We also highlight some of the risks introduced through information systems in the urban environment.
Implementation and Deployment
Designing and deploying smart cities needs experts from multiple fields, including economics, sociology, engineering, ICT, and policy and regulation. Various frameworks describing the architecture of smart cities have been proposed by both industry and academic sources. One of the most widely adapted and adopted models is the reference model proposed by the U.S. National Institute of Standards and Technology. Smart cities are complex systems, often called “systems of systems,” including people, infrastructure, and process components (see Figure 1). Most smart cities models consist of six components: government, economy, mobility, environment, living, and people. The European Parliament Policy Department said in 2014 that 34% of smart cities in Europe have only one such component.
Multiple approaches and methods have been proposed to evaluate smart cities from multiple perspectives, including an urban Internet of Things (IoT) system for smart cities, sustainability, global city performance, future urban environments, urban competitiveness, and resilience. But several fundamental architectural components must be in place to make a city smart.
Essential components. The basic underpinnings of a smart city include five components:
Broadband infrastructure. This infrastructure is pivotal, offering connectivity to citizens, institutions, and organizations. However, today’s Internet lacks the robustness needed to support smart cities’ services and data volume. It includes both wired and wireless networks. Wireless broadband is important for smart cities, especially with the explosive growth of mobile applications and popularity and the connectivity of smart devices;
E-services. The concept of “electronic services” involves using ICT in the provision of services, including sales, customer service, and delivery. The Internet is today the most important way to provide them (such as for tourism, city environment, energy, transport, security, education, and health). A European Union research initiative (called the innovation framework H2020) focuses on developing such e-services; and
Open government data. Open government data (OGD) means data can be used freely, reused, and redistributed by anyone. A multinational initiative to promote worldwide adoption of OGD was launched in 2012 with input from the Microsoft Open Data initiative, Organization for Economic Cooperation and Development, and U.S. Open Data Initiative (http://www.data.gov).
A smart city can be seen as an open data generator. Implementation questions include: How can we efficiently sort and filter the data being produced? Who are the legal owners of the data? And what are the restrictions on the data? Navigating OGD can be related to the open data barriers of task complexity and information quality. With OGD, there is no explanation of the data’s meaning, and discovering the appropriate data is difficult. Data is often duplicated, and data formats and datasets are likewise often too complex for humans and even machines to handle. In 2015, the Spanish standardization normative UNE178301 was published to help cities evaluate the maturity of their own open data projects by relying on five characteristics of their data—political, legal, organizational, technical, and social. OGD will have an enormously positive effect on services offered to citizens, thus improving their daily lives. In this context, linked open data (LOD) could support complex and interdisciplinary data mining analysis.12 LOD is complex because data viewed in isolation may be irrelevant but when aggregated from various sources can yield more meaningful results and fresh insights. LOD is used in such applications as linked data in libraries for creating globally interlinked library data, linked data in biomedicine for creating orthogonal interoperable reference ontologies, and linked government data for improving internal administrative processes.
By leveraging advanced power systems, networking, and communication technologies, a smart city aims to enhance the lives of its citizens and optimize territorial, economic, and environmental resources.
Sustainable infrastructures. The International Electrotechnical Commission (IEC) says cities aiming to develop into smart cities should start with three pillars of sustainability: economic, social, and environmental. One of the first steps in addressing sustainability is to increase resource efficiency in all domains (such as energy, transport, and ICT). An efficient and sustainable ICT infrastructure is essential for managing urban systems development. Adepetu et al.1 explained how an ICT model works and can be used in sustainable city planning. For a sustainable ICT infrastructure, they defined various green performance indicators for ICT resource use, application lifecycle, energy impact, and organizing impact.
E-governance. This component focuses on a government’s performance through the electronic medium to facilitate an efficient, speedy, transparent process for disseminating information to the public and also for performing administration activities. An e-government system consists of three components: government-to-citizen, government-to-business, and government-to-government. E-government allows citizens to fulfill their civic and social responsibilities through a Web portal. A growing number of governments around the world are deploying Web 2.0 technologies, an architecture referred to as “e-government 2.0,” linking citizens, businesses, and government institutions in a seamless network of resources, capabilities, and information exchange.
Fundamental technologies. The design and implementation of smart cities also involves a number of technologies:
Ubiquitous computing. Ubiquitous devices include heterogeneous ones that communicate directly through heterogeneous networks. The UrBan Interactions Research Programd at the University of Oulu, Oulu, Finland, studies urban computing, interaction among urban spaces, humans, information technology, and information.8,18 Ferreira8 described how to build context-aware applications, collect data, and study human behavior. To support a smart and ubiquitous environment, telecommunication infrastructures, Lee13 said, should be enhanced to provide a better understanding of networks, services, users, and users’ devices with various access connections. Lee13 also identified six capabilities and functions of smart ubiquitous networks including context awareness, content awareness, programmability, smart resource management, autonomic network management, and ubiquity.
Big data. Traditional database management tools and data processing applications cannot process such a huge amount of information. Data from multiple sources (such as email messages, video, and text) are distributed in different systems. Copying all of it from each system to a centralized location for processing is impractical for performance reasons. In addition, the data is unstructured. Deploying thousands of sensors and devices in a city poses significant challenges in managing, processing, and interpreting the big data they generate. Big data,10 reflecting such properties as volume, variety, and velocity, is a broad term for complex quantitative data that requires advanced tools and techniques for analyzing and extracting relevant information. Several challenges must be addressed, including capture, storage, search, processing, analysis, and visualization. Also needed is a scalable analytics infrastructure to store, manage, and analyze large volumes of unstructured data. Smart cities can use multiple hardware and software technologies to process the big data being produced, including parallel system architectures (such as cluster-based high-performance systems and cloud platforms), parallel file systems and parallel input/output (such as parallel file systems for big data storage and NoSQL databases for big data), programming (such as low-level programming models, skeletal parallel programming, and generic parallel programming), data management on multilevel memory, and hierarchy task scheduling.15
Networking. Networking technologies enable devices and people to have reliable communications with one another. Several wireless networking technologies, including radio frequency identification (RFID), ZigBee, and Bluetooth, have been deployed, although they are limited by the number of devices they can support, along with their throughput and transmission range. New wireless technologies (such as WiMAX and Long-Term Evolution) are unsuitable due to their high energy consumption. Novel Wi-Fi technology (such as by the IEEE 802.11ah Task Group) could be an efficient solution for smart city services.11 IEEE 802.11ah aims to help design an energy-efficient protocol allowing thousands of indoor and outdoor devices to work in the same area and a transmission range up to 1km at default transmission power of 200mW.11
Smart cities need citizens to be continuously connected—in public places, in public transportation, and at home—in order to share their knowledge and experience.
IoT. One of the main IoT goals is to make the Internet more immersive and pervasive. As a network of highly connected devices, IoT technology works for a range of heterogeneous devices (such as sensors, RFID tags, and smartphones). Multiple forms of communications are possible among such “things” and devices. IoTs must be designed to support a smart city’s vision in terms of size, capability, and functionality, including noise monitoring, traffic congestion, city energy consumption, smart parking meters and regulations, smart lighting, automation, and the salubrity of public buildings.11 They must exploit the most advanced communication technologies, thus supporting added-value services for a city’s administration and citizens.
Cloud computing. Cloud computing enables network access to shared, configurable, reliable computing resources. The cloud is considered a resource environment that is dynamically configured to bring together testbeds, applets, and services in specific instances where people’s social interaction would call for such services;
Service-oriented architectures (SOAs). An SoA is a principle for software structuring based on service. A smart city’s development should focus on SOA-based design architectures to address its challenges. A smart city thus requires a new IT infrastructure, from both a technical and an organizational perspective.
Cybersecurity architectures. Smart cities pose challenges to the security and privacy of citizens and government alike. The security issues associated with the information produced in a smart city extend to relationships among those citizens, as well as their personal safety. Some smart cities are already confronted by identity spoofing, data tampering, eavesdropping, malicious code, and lack of e-services availability. Other related challenges include scalability, mobility, deployment, interoperability (of multiple technologies), legal, resources, and latency. Critical infrastructure needs protection from attacks that could cripple or severely damage a city’s ability to function, from industrial plants to essential services, including access to electricity, water, and gas. Attackers exploiting vulnerabilities in industrial control systems (such as the supervisory control and data acquisition, or SCADA, systems that manage it) can cause significant disruption in service delivery. In vehicular ad hoc networks (VANETs), the standard 1609.2-2013 recommends using pseudonymous authentication for protecting the location privacy of vehicles. Adding layers of security (such as VANETs, SCADA, and mobile networks) in an independent way will make the architecture of a smart city very complex. A smart city thus requires a cybersecurity architecture by design.
Projects and Standardization Efforts
Many cities have piloted their own smart city projects; see Table 1 for specific smart city developments in Asia, Europe, and North America.
Smart cities incorporate digital services as part of a livable and sustainable environment for their citizens. Along with their benefits comes a set of new issues regarding, say, open data and interoperability for policymakers, companies, and citizens alike. Cities need performance-evaluation indicators to measure how much they might possibly improve quality of life and sustainability. Performance evaluation indicators often lack standardization, consistency, or comparability from city to city. A series of international standards is being developed to provide a holistic, integrated approach to sustainable development and resilience under ISO/TC 268 (sustainable cities and communities standard).
ISO 37120 establishes a set of standard performance-evaluation indicators that provide a uniform approach to what is measured and how that measurement is to be undertaken. The International Telecommunications Union defines various key performance indicators for smart sustainable cities through the standard ISO/TR 37150 for fire and emergency response, health, education, safety, transportation, energy (the percentage of a city’s population with authorized electrical service), water (the percentage of a city’s population with a potable water supply service), social equity, technology and innovation (such as number of Internet connections per 100,000 people), CO2 levels and reduction strategies, and buildings (such as energy consumption of residential buildings). Fujitsu has also proposed a performance indicator for ICT in smart cities, using such factors as a city’s environmental impact, the ratio of renewable energy to total energy consumed, and a community’s power-outage frequency rate.
Some type of benchmarking method is pertinent to compare smart cities. Various benchmarking methods were proposed in 2014 for such comparison. For instance, Pires et al.19 analyzed a Portuguese initiative that uses common indicators to benchmark sustainable development across 25 Portuguese cities and municipalities.e The Smarter City assessment tool developed by IBM can be used to measure a city’s performance against indicators for each smart city system. It identifies opportunities for improvement by evaluating component effectiveness, taking into account their degree of modernity, CO2 emissions, and the city’s energy management. This analysis is based on multiple indicators to measure the current situation and the potential for improving a city for the following components of civil society: civic life (degree of modernity, public safety, education, and housing); economic life (business attraction strategy and online services for businesses); mobility and transport (wireless networks and telecommunications infrastructures); energy management (production rate of renewable energy, smart metering, and CO2 emissions); water management (water quality and smart metering); and city services. Another smart city tool, developed by Boyd Cohenf of Universidad del Desarrollo in Chile, includes the environment, mobility, governance, economy, people, and living components (such as health, safety, cultural level, and happiness).
Detecting behavioral anomalies in daily human life is very important for developing smart systems.
Table 2 outlines the benchmarking methods aimed at measuring smart cities from multiple perspectives.
Various organizations and academic institutions have been working on smart city models. As mentioned earlier, the IEC uses three pillars of sustainability—economic, social, and environmental—to develop smart cities. IBM combines instrumentation, interconnection, and intelligence in its smart cities model. Table 3 outlines some of the efforts being undertaken by various organizations.
Challenges and Research Opportunities
Here, we highlight some of the challenges faced by smart cities while exploring research opportunities that need more attention to assist smart city development and adoption.
Challenges. The following are the most noteworthy challenges to be addressed.
Lack of investment. The concept of smart cities reflects strong potential for investment and business opportunities. On the one hand, investment in related projects has grown in recent years, financed by both governments (including municipalities and public research agencies) and private entities (companies and citizens). Navigant Research says investment in smart cities is divided into smart government, smart building, smart transport, and smart utilities. By 2020, $13 billion in funding is expected to establish smart cities all over the world (see Figure 2). Yet according to a 2014 research report on financing models for smart cities, Navigant Research said this infrastructure faces major financial hurdles, including the perceived high risk of investing in innovative solutions, uncertainty of energy price policies, major investment required, long-term delays before reaping profits, and limited capacity for public funding.
Cost. Many cities are committing large budgets to get smarter. For instance, Saudi Arabia is investing U.S.$70 billion in the King Abdullah Economic City (2005–2020) in collaboration with Orange Business Services, Ericsson, Siemens, Cisco, and other companies. In Dubai, 1,000 government services are expected to be “smart” in a few years. In 2015, South Africa began a $7.4 billion smart city projectg to achieve carbon neutrality in public transport by 2025. The bus system in Copenhagen, Denmark, costs €125 million annually. In India, the national government’s annual budget for development of 100 smart citiesh is $1.27 billion, adding 11.5 million homes annually. In the European Union, smart city market projections are expected to exceed $1 trillion by the end of 2016. China’s future smart cities allocations exceed $322 billion for more than 600 cities nationwide.i All these projects demonstrate how substantial is the rate of investment in smart cities. However, if some of the challenges (such as cybersecurity) are not addressed early, the ultimate cost of smart cities will only increase.
High energy consumption. The U.S. Energy Information Administration estimates approximately 21% of the world’s electricity generation was from renewable energy in 2011, with a projected increase to nearly 25% by 2040. The absence of natural resources in the estimation of energy consumption for the rest of the 21st century plays a negative role in smart cities investments (see Figure 3). The future of energy cost and access is uncertain due primarily to their dependence on projected geopolitical, socioeconomic, and demographic scenarios.
Smart citizens. Social dimensions must also be taken into consideration. A city’s “smartness” greatly depends on citizens’ participation in smart city projects, through multiple communication tools (such as a municipality’s Web portal, social networks, and smartphone applications). Smart cities need citizens to be continuously connected—in public places, in public transportation, and at home—in order to share their knowledge and experience. The objective is effective management of natural resources and a higher quality of life for citizens; for example, they can compare their household use of electricity, gas, and water through their smartphones. Adequately maintaining this social dimension is a challenge, though it is a vital aspect of a smart city’s functionality that, exploited correctly, yields dividends for both citizens and the city.
Privacy. Privacy will play a pivotal role in any smart city strategy. Citizens interact with smart city services through their smartphones and computers connected through heterogeneous networks and systems. It is thus imperative smart cities, founded on the use of ICT, be adept at handling important privacy issues (such as eavesdropping and confidentiality). Domingo-Ferrer7 divided privacy into three dimensions: respondent, user, and owner. Martinez-Ballesté et al.16 proposed the concept of citizens’ privacy based on statistical disclosure control, including methods for safeguarding the confidentiality of information about individuals when releasing their data. Examples of such methods are private information retrieval (obtaining database information belonging to someone and hiding it), privacy-preserving data mining (collaboration between entities to get results without sharing all the data), location privacy, anonymity and pseudonyms, privacy in RFID, and privacy in video surveillance.
Cyberattacks. As with any infrastructure, smart cities are prone to cyberattack, and the current attack surface for cities is wide open. IOActive Labs identified several causes of cyberattack: lack of cybersecurity testing, poor or nonexistent security features in connected devices, poor implementation of security features, encryption (outdated and weak encryption algorithms), lack of computer emergency response teams, large and complex attack surfaces, patch deployment issues, insecure legacy systems, lack of cyberattack emergency plans, and denial of service (DoS).
A 2015 workshopj identified several challenges, including vulnerabilities in the transfer of data, physical consequences for cyberattacks, collection and storage of large amounts of data in the cloud, and exploitation of city data by attackers.
Detecting behavioral anomalies in daily human life is very important for developing smart systems. Table 4 outlines some research anomaly-detection frameworks being proposed to detect behavioral anomalies in human daily life in smart-home and smart-grid environments in the context of smart cities. Multiple challenges include characterization of the behaviors of sensor nodes (such as the accuracy of detection, false positive rate, and low computational cost).
Research opportunities. Consider these research opportunities.
IoT management. The IoT needs an efficient, secure architecture that enhances urban data harvesting. As others have noted, ubiquitous and collaborative urban sensing integrated with smart objects can provide an intelligent environment. Otherwise, packet latencies and packet loss are inevitably not controllable. One such proposal is the Mobile Ad hoc Networks (MANET) coordination protocol to opportunistically exploit MANET nodes as mobile relays for the fast collection of urgent data from wireless sensor networks without sacrificing battery lifetime. Simulation results show that their cluster formation protocol is reliable and always delivers over 98% of packets in street and square scenarios. Other issues, including the convergence of IoT and intelligent transportation systems require further investigation.
Data management. Data plays a key role in a smart city. A huge quantity of data will be generated by smart cities; understanding, handling, and treating it will be a challenge. However, mobile phone data can help achieve several smart city objectives. Smartphone data can be used to develop a variety of urban applications. For example, transportation analysis through mobile phone data can be applied for estimating road traffic volume and transport demands. Real-time information from mobile-phone data about the origins of visitors combined with taxis’ Global Positioning System data could help manage transportation resources, as in, say, the public’s future demand for taxis.
A smart city also needs a large amount of data storage, which can be achieved through, say, an electrically tunable metasurface, or an array of nano-antennas.21,22 This technology could affect a range of fields (such as imaging, communication, encryption, and data storage).9 The response of a given smart city to uncertain data is significant, leading to the question of how to measure the robustness of a smart city.
Smart city assessment framework. Livable cities must be monitored through a quality-of-living index, measuring the health, safety, and prosperity in the city. An assessment framework must take into consideration various characteristics, including smart city strategy and the interests of all stakeholders (such as performance evaluators, ICT infrastructures, legal and regulatory policies, services, business models, and sustainability). The objectives of such a framework are to compare the characteristics of different smart cities to identify new challenges, quantify benefits, and evaluate performance.
VANET security. In smart cities, efficient security support is an important requirement of VANETs. One consideration is how to secure them by designing solutions that reduce the likelihood of network attacks or even how to diminish the effect a successful attack could have on them.
Several security challenges persist in the realm of authentication and driver-behavior analysis. A smart city needs lightweight, scalable authentication frameworks that protect drivers from internal and external attackers. The IEEE 1609.2 v2 standard specifies a set of security services (such as certificate authority) for supporting vehicular communications. However, public key infrastructure-based (PKI-based) solutions to verify vehicle authenticity might not lead to a scalable solution. Cross-certification should be defined when countries or cities have multiple root certificate authorities. The authority responsible for misbehavior detection should be incorporated into the PKI system. City governments need to investigate innovative fast, low-cost message-exchange solutions whose communication overheads remain constant as the number of vehicles within communication range increases. On the other hand, to ensure vehicle privacy and anonymity, pseudonym change (pseudonymous certificates with removed identifiable information are used in PKI) strategies (such as fixed time change, random change, and density-based change) should be designed and tested on a large scale, as in a smart city environment. Desired security properties include lightweight authentication, security architecture scalability, and resilience against malicious nodes and DoS attacks.
Elliptic curve cryptography is considered the most trusted solution for providing security on resource-constrained devices and embedded systems.
Improving photovoltaic cells. Achieving a sustainable energy source in a smart city must include renewable energy. Solar technology has made significant strides (such as photovoltaic cells that convert light energy into electricity) in the past decade. Still, more efficient solar-energy-harvesting techniques are needed to improve solar cells. The vast majority of today’s solar cells absorb light through a thin film of cadmium telluride composed of two layers of glass. The benefits of thin-film solar cells include low production cost and ease of fabrication. New design approaches emerged in 2014 based on nanophotonics, whereby nano-antennas are exploited for guiding and localizing light at the nanoscale. Such designs promise to improve the absorption process in photovoltaic devices, thus enabling a considerable reduction in the physical thickness of solar-absorber layers and paving the way for new solar-cell designs. In this context, designing new micro- and nano-antennas for improving light absorption is important, and dimer nanostructures, or structures composed of two nanoparticles turn out to be good candidates.2
Smart city enablers. Technology advances are helping create a market for smart city products and solutions, but smart cities need efficiency and sustainability. Angelidou4 proposed the conjuncture of four forces: urban futures, technologies, applications, and innovation economy. To develop, smart cities must leverage technological advancements and development of knowledge and innovation networks.4 In addition, to create a smart city, Amaba3 proposed integrating multimedia, human factors, user-centered system methodology, and design principles. Smart cities can provide solutions to many sustainability problems, but their cohesive development requires an effective policy to be in place as part of any solution.
Information system risks. In a smart city, everything is interconnected, including the public water system, traffic control, public transportation, and critical infrastructure. Each one involves its own vulnerabilities. Although a smart city is a complex system, its interconnected nature means a single vulnerability could greatly affect citizens’ security; for example, an attacker might be able to connect to the electric power system to gain access to the network and alter public transportation to potentially paralyze intelligent transportation systems, with thousands of passengers on board at rush hour. The attacker could also launch false alarms and modify traffic lights and controllers. Finding workable solutions is critical; otherwise the public will not trust smart city projects or deem them viable. Devices (such as smartphones) used in the IoT depend on security features (such as the ability to do secure email, secure Web browsing, and other transactions). These devices need efficient, secure implementations of all these features in a smart city. In this context, elliptic curve cryptography (ECC) is considered the most trusted solution for providing security on resource-constrained devices and embedded systems. ECC uses public key cryptography and is based on the algebraic structure of elliptic curves over finite fields. ECC advantages include the ability to provide the same level of security as other cryptographic algorithms but with smaller keys, low memory requirements and computational overheads, and much faster computations.
The scientific community must address these cybersecurity projects. Unresolved challenges and opportunities for participation involve DoS-attack detection for distribution systems, cryptographic countermeasures, and authentication in IoT and critical infrastructure, as well as in key management.
Conclusion
The strong interest by municipal and local governments worldwide in smart cities stems from their ability to improve their citizens’ quality of life. Here, we described some of the basic concepts of smart cities, identifying challenges and future research opportunities to enable large-scale deployment of smart cities. Developers, architects, and designers should now focus on aspects of IoT management, data management, smart city assessment, VANET security, and renewable technologies (such as solar power). We underscore when designing smart cities, security and privacy remain considerable challenges that demand proactive solutions. We hope to see smart city developers, architects, and designers provide scalable, cost-effective solutions to address them in the future.
Acknowledgments
We express our gratitude to Teodora Sanislav, Nils Walravens, and Lyes Khoukhi for their comments and suggestions on early drafts of this article, helping improve its content, quality, and presentation. We would also like to thank the reviewers for their valuable feedback and reviews.
Figures
Figure 2. Smart city infrastructure investments by industry, 2014–2023.
Figure 3. Estimating future energy consumption.
Figure. An operator checks the electric power control panel for the smart city project in Kashiwa, Japan, on July 7, 2014.
Join the Discussion (0)
Become a Member or Sign In to Post a Comment