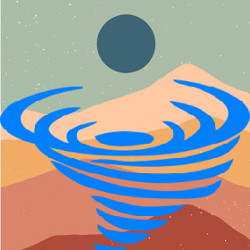
An emerging discourse in both popular and academic literature now exists regarding the potentially game-changing impact of quantum computing and quantum communications.6,18 Perceived returns on investment in quantum computing and its potential to disrupt the current classical digital-computing landscape has intensified competition amongst the so-called big tech companies and selected high-performing start-ups to deliver a functional quantum computer.15,17 While several software tools have been made available either freely or on an open source basis, most research and development on hardware by big tech companies and start-ups remains proprietary. This makes it challenging to realistically assess available quantum-computing capabilities and to distinguish the hype from market realities.1,23 Despite increasing, potentially unrealistic expectations and timelines associated with quantum computing,14,38 there appear to be a limited number of studies assessing the technology landscape from a market perspective as of 2021–2022.
Key Insights
- Quantum hardware and software currently being developed are mainly aimed at solving computational problems using a fundamentally different paradigm than a classical computer.
- Expectations about what quantum computing can or cannot deliver must be independent of the capabilities of classical computers.
- Prior to the widespread commercial availability of universal quantum computing, a hybrid computing approach is likely to prevail, one which draws on classical computers and advancements in quantum-computing hardware and software.
- While many of quantum computing’s promised capabilities could be revolutionary, the realization of this promise requires breakthroughs in several areas, including improvements in the quality of qubits, error correction, and a demonstrable set of practical applications.
This article aims to assess quantum-computing developments with a focus on the market readiness of developed technologies and the reported levels of investment in them by the following key players: big tech companies, start-ups, and nation-states. It draws on desk research to present this market assessment. The discussion in this article is intended to be an initial, exploratory review of the current state-of-play rather than a comprehensive investigation of the market players. The aim is to offer a more informed understanding of the current capabilities of quantum-computing technologies and the implications they hold for computing’s future. The findings are expected to be of interest to quantum-computing researchers; policymakers; market players, such as venture-capital investors, technology companies, and nation-states investing resources in quantum computing; and those who follow emerging technology trends.
This article begins with a discussion of the research approach, including its limitations, followed by a description of key terms and an analysis on the market readiness of quantum-computing hardware and software. Next is a discussion on the reported levels of investment in quantum-computing technologies, followed by considerations of the implications for the future of the quantum-computing landscape. The article concludes with reflections on the findings.
Research Approach
To understand the current state of quantum-computing technology developments, this article used a highly targeted form of literature review based on a scan of available literature via Google Scholar and Google. As part of the initial scan, literature searches covered peer-reviewed journal articles and conference papers on Google Scholar using combinations of the following search terms: “quantum computing,” “market readiness,” “level of investment,” “technology readiness,” “cost of development,” “total addressable market,” “market size,” “*big tech*,” “start-up*,” and “start up*.”
Grey literature was excluded from initial searches conducted on Google Scholar. However, the initial scan on Google Scholar for these terms primarily yielded technical literature on quantum computing. Consequently, the search criterion was broadened to include grey literature (technology consulting and policy reports, blogs, technology magazines, and news articles). The second round of searches were conducted on Google instead of Google Scholar. Search results after 2010 were prioritized and only articles published in English were considered as part of both rounds of literature scans.
For the literature identified during this scan of Google and Google Scholar search results, the abstracts and executive summaries were reviewed at a high level. Forty-eight articles were short-listed based on their relevance to this article’s goals, and these were reviewed in detail to categorize the findings based on the following relevant parameters: definitions, approaches to quantum-computing, market readiness of the technology, reported levels of investment, and implications for the future development of quantum-computing technologies. Information extracted as part of the review of the final short list of articles forms the basis of the discussion presented in the rest of this article. Forty-three additional articles were identified by scanning relevant references in the short-listed articles (that is, snowballing) to cross-check and triangulate the findings. The articles most relevant to the narrative in this article are cited and included in the References section.
Given the highly targeted nature of the literature scan, some caveats to the findings provided in this article must be considered. The scan relied on information in the public domain and resulted in primarily qualitative literature being identified. The data sources were secondary in nature, and commercially available quantitative information on quantum-computing developments was excluded due to the cost of acquiring the data. Therefore, the resulting analysis in subsequent sections is exploratory rather than a comprehensive coverage of available literature.
Although there is a growing body of literature focused on the application of quantum-computing technologies to the field of security and encryption, this article focuses on general-purpose quantum computing. Extensive coverage of topics related to quantum encryption and quantum security methods is out of this article’s scope. Literature on quantum communications—that is, the application of quantum physics to information processing, networks, and communications—is also excluded from the analysis. This is to facilitate a calibrated discussion on the state of quantum-computing technology developments.
This article focuses on big tech companies, start-ups, and nation-states. Though the literature identifies market players in investment banking, automobile manufacturing, oil and gas production, insurance, and multinational retail banking as key investors in the technologies, they are excluded from the discussion. While China is a leading investor in quantum-computing technologies along with the U.S., English-language literature appears to mainly target developments in North America, Europe, and other countries in the Asia-Pacific region, such as Singapore and Australia.15 As a result, this article presents only a partial picture of the market developments in China, focusing on the following Chinese big tech companies: Alibaba, Baidu, and Tencent.
One of the main challenges facing the small-scale quantum computers currently in use is that when the hardware is scaled up, the hardware errors also scale up exponentially. The quality of qubits, including their ability to retain the qubit state in a persistent manner, is unproven.16,17 Also a key contributing factor to the errors is decoherence, in which qubits interact with the external environment, change their quantum states, and lose information stored in the qubits.28 If breakthroughs in error correction, noise reduction, or quantum transistors occur earlier than currently forecast, the timelines for mainstream adoption of technology can be expected to change significantly.
The findings in this article are a snapshot of the state-of-play as of 2021–2022. They would need to be reassessed in the case of unforeseen breakthroughs.
Description of Key Terms
This section provides a brief description of key terms related to the discussion on quantum computing:
Quantum bit. A quantum bit (qubit) is the quantum-computing equivalent of the bit in classical computing. To understand the difference, it helps to visualize a classical bit and a qubit as plotted on a 3D sphere.18 A classical bit has only two values, 0 or 1, and therefore can only take the value of the north or the south pole on this sphere. In contrast, a qubit can take a value of any point of the surface on the sphere, including every possible pair of latitude/longitude measurements. The qubit can also simultaneously take intermediate positions between 0 and 1. As a result, for n qubits, the same operation can be performed on all the possible combinations of 0 and 1 for all the qubits — that is, 2n combinations are possible.18
Quantum algorithm. A quantum algorithm is a set of instructions solving a problem that can be performed on a quantum computer.4
Quantum supremacy. Quantum supremacy (also quantum primacy or quantum advantage) is achieved when a quantum device is proven to be able to carry out a task that a classical computer would find impossible or would take too long to complete and is unlikely to be overturned by algorithmic or hardware improvements to classical computers.26
Universal quantum computer. A universal quantum computer is identified as one endowed with the full set of classical computer capabilities using quantum algorithms and hardware.10
NMR, ion-traps, and other quantum techniques. Existing proposals for building quantum computers focus on using ion traps, nuclear magnetic resonance (NMR), optical/photonic, and solid-state techniques.33 Of these, NMR and ion-trap technologies are the most advanced, with optical and solid-state techniques holding promise for the future.33 These approaches all suffer from quantum noise and scaling problems to impede progress beyond tens of qubits and into the hundreds of qubits.33 Experts suggest that for quantum computers to be useful in solving real-world problems, the devices need to scale up to millions of qubits.25
NISQ computers. Current-day quantum computers are referred to as noisy intermediate-scale quantum (NISQ) computers, signifying that they have few qubits, limited gate depths, and short coherence times.18
One of the main challenges facing small-scale quantum computers in use is that when the hardware is scaled up, hardware errors also scale up.
Big tech. This term refers to the world’s largest information technology companies in terms of market availability; revenue; operational footprint, and tangible/intangible socioeconomic, political, and legal influence. In this article, big tech refers to the following companies: Alibaba, Amazon, Apple, Baidu, Facebook (Meta), Google (Alphabet), IBM, Intel, Microsoft, and Tencent.
Start-up. This describes a company or project undertaken by an entrepreneur or a group of entrepreneurs to develop a viable business model based on developing quantum-computing software or hardware capabilities either in conjunction with or independent of big tech companies.
Market Readiness of Current-Day Quantum-Computing Technologies
As of 2021, a universal quantum computer capable of performing operations equivalent to current computers, smartphones, and other smart devices remains decades away. Most notable breakthroughs reported in the literature have been about hardware devices, indicating that the focus of quantum-computing investments has been on hardware development.
The following discussion takes this into consideration, using two parameters to assess the market readiness of quantum-computing hardware and software: published results or public demonstrations of the technology, and the extent to which the hardware or software is accessible and available.
In the reviewed literature, the atomic clock capable of split-second precision is commonly cited as the earliest demonstrable example of the technology.2,31 Other notable examples of quantum-computing hardware from the 1990s include:
- A simple, two-qubit NMR quantum computer built in 1997 by researchers from IBM Almaden Research Center in San Jose, Massachusetts Institute of Technology in Cambridge, and the University of California.20
- Three-qubit NMR quantum computers demonstrated in 1998 by researchers at Los Alamos National Laboratory in New Mexico.20
- A simple, two-qubit quantum computer built by researchers at the University of Oxford in 1999.20
The use of quantum sensors in devices such as atomic clocks and gravi-meters is considered a practical, useful contribution of the technology.2,31 In particular, quantum sensing is expected to become a key component over the next five to seven years in quantum positioning navigation and timing (PNT) devices and quantum radar technologies.2
Market readiness of quantum-computing hardware. When hardware developments in quantum computing are considered, several technology demonstrations have been made by big tech companies, such as Google, IBM, and Intel, as well as academic researchers.
Notable examples include:
- In early 2015, Google demonstrated a linear array of nine qubits in operation.36
- In late 2015, demonstrations by Veldhorst et al.39 and Hensen et al.22 highlighted breakthroughs in solid-state spin qubits, which potentially make quantum computing more practical.36
- In 2016, Google simulated a hydrogen molecule with a nine-qubit quantum computer.25
- In 2017, Intel reached 17 qubits, and IBM built a 50-qubit chip that could maintain its quantum state for 90 μs.25
- In 2018, Google unveiled Bristle-cone, its 72-qubit processor.25
- In 2019, IBM launched its first commercial quantum computer—the 20-qubit IBM Q System One.25
- As of 2019, IBM and Canadian firm D-Wave Systems were reported to sell access to quantum-enhanced calculators.3 Biamonte et al.3 also reported that Google, Microsoft, and Intel had plans for similar offerings in three to five years.
- As of 2020, IBM had built Q System One, a 53-qubit quantum computer for which it was reported to have reached sales agreements with Germany and Japan.32
Each of these demonstrations indicate incremental growth in the qubit capabilities of available quantum machines. Most of these quantum machines have at best a few dozen qubits.9 Due to the computation-destroying noise inherent in current technology, most of these demonstrations have limited practical uses.9 For example, although Google’s biggest quantum computer had 72 qubits as of 2019, a general-purpose quantum computer will require approximately one million qubits to be functionally useful.15
A general-purpose quantum computer will require approximately one million qubits to be functionally useful.
An important breakthrough in the technology’s market readiness was reported in 2019 by Google, which claimed to have reached quantum supremacy.21 Google contended that its quantum computer (Sycamore) performed a calculation in 200 seconds that would take a classical supercomputer 10,000 years.15,17,21 IBM, however, questioned this claim, arguing that a classical supercomputer could perform the calculation in two-and-a-half days.12,15,17,18 Setting aside the performance debates, from the perspective of large-scale, general-purpose quantum computing, Google’s Sycamore represents an important step, since it can detect and fix computational errors.35 However, Sycamore’s current system generates more errors than it solves.35
Despite the challenges of error correction and decoherence, IBM announced a road map in September 2020 for the development of its quantum computers, including its goal to build a quantum computer with 1,000 qubits by 2023.2 Google has also indicated plans to build a million-qubit quantum computer by 2029.2 Intel is reportedly readying a superconducting quantum computer.18
When considering hardware built by start-ups in the quantum-computing space, Canada-based D-Wave is often cited as an outlier.4,25,36 D-Wave has been selling commercial quantum computers since the late 1990s and claims to have several thousand “annealing qubits” in its devices.25 However, annealing qubits are only useful in certain types of problems; therefore, quantum annealers are not general-purpose computers.25 Given the current state of development of the technologies, quantum annealers are energy intensive and very expensive. For example, the D-Wave 2XTM computer, a quantum annealer built in 2015, had 1,000 qubits, consumed 25 kW of power, and cost roughly 15 million USD.36
Other notable examples of quantum-computing hardware start-ups include IonQ, which plans to produce a device roughly the size of an Xbox videogame console by 2023.23 One source cites Rigetti and IonQ as having developed integrated chips suitable for quantum computing.18 PsiQuantum, a U.K.-based start-up, expects to be building fault-tolerant quantum computers with fully manufactured components capable of scaling to a million or more qubits by the mid-2020s.23 For this, PsiQuantum has partnered with semiconductor manufacturer Global-Foundries to build machines that are comparable in size to supercomputers or data centers and will be accessible to users remotely.23,37 Rigetti Computing, based in Berkeley, CA has built its own 31-qubit machine.27 Amongst companies providing specialized hardware capabilities, Janis Research Company is the only U.S.-based commercial manufacturer providing cooling solutions for superconducting quantum computers, and the Swiss Federal Institute of Technology (ETH)-led Quantum Engineering Initiative (QEI) provides analog and digital control electronics and device fabrication.18
When general-purpose quantum-computing hardware is considered, developments in China are also significant in assessing the technology’s market readiness. The Chinese government has decided to allocate 10 billion USD for the country’s National Laboratory for Quantum Information Sciences.40 In 2020, a team of researchers from the University of Science and Technology of China reported having achieved quantum supremacy by completing Gaussian boson sampling (a problem considered intractable for classical computers) in just 200 s with a device named “Jiuzhang.”12,26,40 The research team behind Jiuzhang suggested that the same calculation would take the “Fugaku” (a super-computer built by Fujitsu at the Riken Center for Computational Science in Kobe, Japan) 600 million years to complete.26 The team argued that when the metrics of performance around Gaussian boson sampling were considered, Jiuzhang was up to 10 billion times faster than Google’s Sycamore.40 Another Chinese quantum computer, “Zuchongzhi,” is a two-dimensional programmable computer composed of 66 functional qubits, which has been reported to perform random quantum circuit sampling (another reportedly intractable problem for classical computers).12
Market readiness of quantum-computing software. An important challenge facing the development of quantum-computing software is that current hardware is highly targeted in terms of functionality—that is, a general-purpose quantum computer does not exist. This results in a paradoxical scenario where the software currently being written is for hardware capabilities which are not yet fully available.20
When the software developed by big tech is considered, Microsoft, IBM, Baidu, and Google have all created tools. These tools—Q# (Microsoft), Qiskit (IBM), QCompute (Baidu), and Cirq (Google)—are primarily based on the Python programming language and include development environments with supporting documentation for software programmers.27 Amazon, Microsoft, IBM, Alibaba, and Baidu have also started offering cloud-computing services with back-end quantum-computing resources in limited preview mode for developers.25,32,34,40
Starting in 2019, Amazon Web Services (AWS) began offering researchers and developers its Amazon Braket quantum-computing development environment in the cloud.32,40 Braket is available in an early preview with quantum computers from D-Wave, IonQ, and Rigetti as the back ends.32 Amazon is reported to be exploring mass-produced quantum computers through its new Center for Quantum Computing.32 Amazon is also said to be analyzing some of the leading startups in the sector for either future partnerships or acquisitions.40
Microsoft’s tools take the form of a quantum development kit (QDK), containing code libraries, a debugger, and a resource estimator.27 Microsoft has also developed Azure Quantum, a cloud-computing service leveraging IONQ’s trapped-ion quantum computer, superconducting qubits being developed by Connecticut-based Quantum Circuits Incorporated (QCI), and Honeywell’s quantum computer as the back ends.25,32 This service is currently available in private preview, though Microsoft says it plans to build its own quantum-computing hardware.32 Azure is second only to AWS in cloud computing as of 2021–2022;32 therefore, Microsoft’s Azure Quantum is a potential challenger to Amazon’s likely leading position in quantum cloud services.40 Microsoft has also developed LIQUi|>, a language-integrated quantum operations simulator platform, in which a quantum algorithm written in the form of a high-level program can be translated into low-level machine instructions for a quantum device.31 It includes a compiler, optimizers, translators, various simulators, and examples for developers.31
Along with Microsoft, IBM was one of the first big tech companies offering public access to an experimental quantum-computing platform.36 In 2016, IBM offered access to IBM Quantum Experience, which enabled cloud-based access to a 5-qubit quantum computer virtual lab for researchers and students.36 IBM also offered access to the IBM Q premium service, for which it was reported to be working with more than 100 companies, including ExxonMobil, Barclays, and Samsung, on practical applications.25 One source identifies IBM as an overall leader in the field with a full complement of hardware and software in addition to the most assigned patents.40
Among Chinese big tech companies, Alibaba appears to lead Baidu and Tencent when quantum-computing investments originating in China are considered.11 In 2018, Alibaba’s cloud service subsidiary Aliyun (Alibaba Cloud) and the Chinese Academy of Sciences jointly launched an 11-qubit quantum-computing cloud service.34 Alibaba is reportedly developing quantum processers at its 15 billion USD science and technology research center.40 Baidu has declared its interest in building a hardware-agnostic software stack with quantum artificial intelligence (AI), quantum algorithms, and quantum architecture as its three priority areas.34 In 2020, Baidu announced Quantum Leaf, which it describes as China’s first cloud native quantum-computing platform.34 Tencent has created a Tencent Quantum Lab (tasked with building a quantum-computing cloud) as part of its planned 500 billion yuan (70 billion USD) expansion into AI, blockchain, supercomputing centers, operating systems for Internet of Things, 5G networks, and quantum computing.29
In addition to big tech efforts, several start-ups have released software development kits (SDKs) for quantum computing. Notable examples include Rigetti Computing’s quantum SDK, Forest, which includes a Python library called pyQuil.27 UK-based Cambridge Quantum Computing (now Quantinuum, part of the Honeywell Group) has launched TKET, along with an associated pytket library.27 Companies such as QxBranch and 1Qbit act as intermediaries between quantum experts and industry, examining whether and how quantum methods might improve a firm’s business.31 Services offered by such firms include optimizing trading strategies or supply chains or monitoring network activity to spot cyber-attacks.31 Other notable software examples include Silq, a language released in 2020 by a team at ETH in Zurich;27 QuTip, open source software packages funded by a number of research organizations in Asia;31 and Quantum Algorithm Zoo at the National Institute of Standards and Technology in Maryland, which is a comprehensive collection of known quantum-computing algorithms.31 QuTip uses Python to make programming quantum computing easier and more accessible using high-level languages.36
Reported Levels of Investment in Quantum-Computing Technology
As of 2021, based on the available information on reported levels of investment in quantum computing, the following discussion covers two strands of investment activity:
- Investments by nation-states into research and development at universities, public-sector research organizations, and industry.
- Private-sector investment, including investments by big tech companies and other private-sector operators, and investments in start-ups by venture-capital companies.
National and supranational funding bodies appear to be the main investors in quantum-computing research.31 Given the security implications of quantum computing, defense departments in many nation-states appear to be the main drivers of funding.31 Although many big tech companies are also reported to be heavily investing in quantum computing, very few appear to have publicly disclosed the amounts involved.15 When venture-capital funding activity is considered, North America is the leader.
Investments by nation-states. Among the nations reported to be investing in quantum computing, the U.S., Canada, European Union (EU), U.K., China, Russia, and Australia are the leaders.3,36 In the North American context, the U.S. Innovation and Competition Act, a 250 billion-USD initiative, has designated quantum information science and technology as one of 10 key focus areas for the National Science Foundation.5 The U.S. National Quantum Initiative Act provides 1.2 billion USD to promote quantum information science over a five-year period.8 As part of this initiative, five new National Quantum Information Science Research centers have been created by U.S. Department of Energy researchers—with participation from academia, U.S. national labs, and industry—to help catalyze quantum information science research.8 In the 2021 budget, the U.S. federal government strengthened this funding with other initiatives, including 237 million USD.19 As part of the Canada First Research Excellence Fund, the University of Waterloo, one of the major North American research hubs, was awarded 76.3 million CAD.36
In 2018, the EU launched billion-dollar investment programs, including the 10-year Quantum Technologies Flagship program.3,31 In 2020, Germany also announced a 2 billion-EUR investment in quantum technology research and development over the next five years, in addition to the European Commission’s 1 billion-EUR fund for quantum technologies.19 The U.K.’s total public and private investment in quantum technology research and development since 2014 is reported to have exceeded 1 billion USD.3 The U.K. also launched a program worth 270 million pounds (337 million USD) in 2017.31
In 2019, China declared its intent to open the world’s largest quantum research laboratory in 2020 at a cost of 10 billion USD.3 The Chinese government reportedly spends at least 2.5 billion USD a year on quantum research.19 Russia is reportedly coordinating private and government research and development of quantum technology as part of large projects that are expected to receive up to 300 million USD in the first phase.3
Other notable investments include the Australian government’s 2016 investment of 46 million AUD into a quantum-computing program based at the University of New South Wales,36 including the ARC Centre of Excellence for Quantum Computation and Communication Technology (CQC2T), which was awarded 33.7 million AUD. Additionally, the University of Queensland’s Centre for Engineered Quantum Systems (EQuS) received 31.9 million AUD for a duration of seven years.36 India and South Korea have also declared their intention to invest tens of millions of dollars per year.3
Private-sector investments. Although most big tech companies (with the notable exception of Apple and Facebook) have announced quantum-computing initiatives, none appear to report actual levels of investment as part of their annual reports. The evidence suggesting that big tech companies are investing millions of dollars in quantum computing research and development is, therefore, mainly anecdotal. Some big tech companies, including IBM, Microsoft, and Intel, along with other industry behemoths such as Applied Materials and Lockheed Martin, have been reported as participating in the new National Quantum Information Science Research centers created as part of the U.S. National Quantum Initiative Act.8
Unlike the big tech companies however, there is significant evidence highlighting the levels of venture-capital funding in start-ups working on quantum-computing hardware and software. More than 80 percent of these investments have been allocated to quantum-computing hardware or its components.15 Start-ups focused on software and applications are likely to be the next growth area.
In 2015, McKinsey reported that a combined investment of 1.5 billion USD had been made worldwide on quantum-technology research.31 Private-sector investments in quantum computing are projected to grow exponentially in the years ahead. Globally, equity investments in quantum computing were reported to have tripled in 2020 and 2021.37 Some sources have projected that the investments in quantum computing will grow from 260 million USD in 2020 to 9.1 billion USD by the end of the decade.32 Honeywell anticipates that quantum technologies will lead to a 1 trillion USD industry in the decades ahead.6
North America is a world leader in attracting venture-capital funding, and it also dominates private quantum investment.15 These investments are not restricted to Silicon Valley. Firms in Canada have attracted 243 million USD, resulting in an ecosystem to support quantum companies around academic hubs in Waterloo and Toronto.15 As of 2019, D-Wave Systems was reported to have raised 177 million USD.15
By late 2021, venture-capital investors had reportedly funded more than 180 quantum-computing companies globally since 2015, with the cumulative investments exceeding 2.4 billion USD.37 The number of venture-capital deals in the North American market rose 46% in 2020 over the previous year, despite the total amount raised in the quantum-computing sector falling by 12% to 365 million USD.40 Although the financial value of some of these investments remains undisclosed, Nature‘s analysis suggests that in 2017 and 2018, companies received at least 450 million USD in private funding,15 more than four times the 104 million USD investments disclosed in 2015 and 2016.15 The number of start-ups being funded due to advances in quantum-computing technology is also likely to increase in the years ahead.40
Notable start-ups reported to have each raised tens of millions of dollars include Zapata Computing (Cambridge, Massachusetts, USA), 1QBit (Vancouver, Canada), and U.K.-based Cambridge Quantum Computing.15 Chinese firms QuantumCTek (in Heifei) and Qasky (in Wuhu), leaders in the field, have raised significant private funding which has not been disclosed.15
Implications for the Future of the Quantum-Computing Landscape
This article has focused on the market readiness of quantum-computing technologies when correlated with the reported levels of investment. Based on this evidence, some key implications can be identified for the various stakeholders — researchers, technology companies, investors, nation-states, and policymakers — interested in the technology:
Given the security implications of quantum computing, defense departments in many nation-states appear to be the main drivers of funding.
Projections and predictions. Any current-day assessments on quantum computing’s market size, transformative effect on various sectors, valuations of its impact, and predictions on mainstream availability of the technology are early, optimistic projections. The technology’s actual addressable market in practice is highly limited as of 2021–2022.37
Talent retention. Since quantum-computing technologies are in the early stages of development, some sources suggest that there is a risk of research talent moving away to start-ups too early. This may fragment the market and limit the potential breakthroughs the research could yield.3,30 Given the growth in patent activity by start-ups, whether the transition of publicly funded research talent to start-ups is hindering fundamental research in the field is also up for debate.3 For example, one source states that due to issues of scaling up technology development in the U.K., the U.K.’s best-known quantum-computing scientists have already moved to the Silicon Valley.30
Impact on computing. Although quantum-computing technologies are still developing, the development of algorithms for quantum computers has led to advances in solutions to large-scale combinatorics problems on existing classical computers.7 This suggests that developments in quantum computing are likely to prove complementary and may help augment and upscale the current capabilities of classical computers.
Drivers and adopters. Given the potential capabilities of quantum computing to process raw, complex datasets at a scale and pace unmatched by classical computers, early adopters are likely to be industries in which data, particularly big data and data analytics, plays a crucial role. This suggests that industries where data is a crucial ingredient, such as healthcare, finance, commerce, communications, security, cybersecurity and cryptography, energy, and space exploration, will be the main drivers of near-term investment in the technology.8 Understanding the role of data will be crucial to any analyses of the next stage of market developments.
Funding prospects. Due to the hype surrounding the technology, there is a risk that quantum-computing research may suffer the same fate as AI research did in the 1980s, resulting in a quantum equivalent of the AI winter. Experts warn that unrealistic expectations of possible breakthroughs could result in research funding being prioritized to address other immediate-term technology research, with research talent moving away before full-scale machines can be built.9,15
Deployment approaches. Based on the state of quantum-hardware capabilities circa 2016, one source estimated that constructing a full-fledged universal quantum computer would cost around 10 billion USD, the equivalent of a next-generation chip fab at Intel.10 As a result, it is highly likely that instead of a single, universal quantum-computing platform, ones which rely on different approaches or hybrid uses of quantum and classical platforms may prevail in the interim depending on their efficacy for different tasks.16
Outcomes and expectations. Compared to classical computers, quantum computers draw on the unpredictable attributes of quantum particles and, therefore, may be more suited to a different, narrower range of outcomes, such as simulating systems dominated by quantum effects.23 The quantum hardware and software being developed by big tech and start-ups is mainly aimed at solving computational problems using a fundamentally different paradigm than a classical computer.21 Expectations of what quantum computing can or cannot deliver must be independent of the capabilities of classical computers.
Role of standards. The potential exists for industry and public-sector stakeholders to start discussing the role of technical standards and codes of conduct as regulatory tools for quantum computers, without undermining market-led innovation.24 At an early stage, these discussions could be industry-led and focus on the security concerns posed by quantum computers in the form of quantum cyber-attacks, including the use of postquantum cryptography.24
First applications. Given the known limitations of the technology in terms of its need for error correction, uncertain quality of qubits, and the challenges in managing decoherence (to name a few), the first market-ready applications of real quantum computers are likely to be discrete, focused on specific uses or outcomes such as verifying random numbers.25,31 Related to the niche hardware capabilities of quantum computers, the focus on software and algorithm development is also likely to shift to smaller quantum machines, which are expected to be available in the near future.31
Developments in quantum computing are likely to prove complementary and may help augment current capabilities of classical computers.
Development trajectory. Although most of the reported funding for quantum-computing research has been provided by nation-states and public-sector authorities, most of the available quantum-computing capabilities on the market are through private technology firms (either big tech or start-ups). In particular, access to quantum-computing hardware is available almost exclusively through private technology firms.27 When contrasted with classical computers in a comparable stage of development (1940s and early 1950s),13 this suggests a different, more market-led trajectory for the development, deployment, and adoption of quantum computers.
Access mechanisms. Most of the notable access to quantum-computing capabilities is in the form of cloud offerings from big tech companies such as Amazon, Microsoft, IBM, Alibaba, or Baidu.25,32,34,40 Most private technology firms (either big tech or start-ups) have developed SDKs or application programming interfaces (APIs) to access the hardware capabilities in private preview mode mainly on a subscription basis. It is quite likely that until the capabilities of quantum computers exceed their current-day niche uses, cloud computing will remain the prevalent form of mainstream access to quantum computers.
Reflections on the Findings
This article highlights that although much of the promised capabilities of quantum computing could revolutionize the field, further breakthroughs in quantum-computing hardware and software are needed to realize this promise. While some hardware capabilities have been demonstrated by companies such as D-Wave, Google, and IBM, they have yet to provide definitive timelines for commercial availability of a quantum computer. Start-ups such as Rigetti and IonQ have reportedly developed integrated chips suitable for quantum computing with Intel readying a superconducting quantum computer.
On the software front, offerings from Google, IBM, Microsoft, Baidu, and start-ups such as Rigetti and Quantinuum have been in the form of APIs, SDKs, simulations, and quantum machine-learning capabilities. Companies such as Amazon, Microsoft, IBM, Alibaba, and Baidu have begun offering cloud services with the prospect of quantum-computing capabilities being available on demand. However, most of these services are currently available in private preview mode. Quantum computers built by companies such as Honeywell, IonQ, and QCI appear to have been at the core of the cloud services offered by Amazon, Microsoft, and IBM.
Efforts by Chinese big tech, such as Alibaba, Baidu, and Tencent, indicate an increasingly competitive market-place with developments dominated by advances being made by organizations headquartered in the U.S. and China. On the one hand, Google has indicated that it wants to build a useful quantum-computing offering by the end of the decade. On the other hand, critics highlight challenges in error correction, decoherence, and potential niche status of quantum computing to argue that the transformative capabilities of the technology are being overstated based on current development status.
When the overall market readiness of the software and hardware powered by quantum computing and the reported levels of investment in the underlying technologies is considered, a complex picture of raw potential, promising early developments, and misleading hype emerges. Available evidence suggests that a demonstrable universal quantum computer is at least a decade away. It may be too early to speculate about widespread commercial availability of universal quantum-computing capabilities. In the interim, a hybrid computing approach drawing on classical computers and advancements in quantum-computing hardware and software is likely to prevail. Due to the technology’s disruptive potential, interest in funding fundamental research in quantum computing and commercial investments in hardware and software development is likely to persist in the near term. However, based on the current state-of-play, making any definitive assessments on timeframes for mainstream adoption and deployment of general-purpose quantum-computing capabilities remains challenging.
Join the Discussion (0)
Become a Member or Sign In to Post a Comment