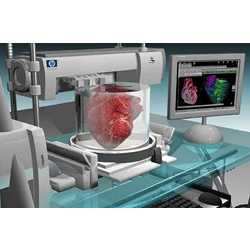
As far back as the late 1990s, Dr. Stuart Williams recalls feeling strongly that researchers needed to find a way to rebuild heart tissue from a patient’s own cells. The work is critical, as wait times for heart transplants can be long. Thus began a path that would lead to the concept of a total "bioficial" heart.
Williams, director of the Bioficial Organs Program in the Cardiovascular Innovation Institute (CII) at the University of Louisville, was approached by the U.S. Defense Department’s Defense Advanced Research Projects Agency (DARPA) in the 1990s, when he was working at the University of Arizona. Williams was already working on ways of taking cells from fat and moving them to other parts of the body, and had built a biological architecture tool (BAT), sometimes referred to as a BioAssembly tool. DARPA asked Williams and his team to collaborate with an Oklahoma-based company called Sciperio that was building three-dimentional (3D) printers that used a paste as their raw material, under the assumption that together they could come up with a type of cell-containing paste that the printers could use.
"We decided to make a bio ink … out of collagen, which is the extracellular glue that holds cells together,’’ Williams says. That led to his eventual goal of building a total bioficial heart, "not from plastic and metal, but from a patient’s own cells."
"Bioficial" refers to the combination of biology and artificial parts.
Three-dimensional printing, also known as additive manufacturing, dates back as far as the 1800s, when it was first used to show the depth of mountains and terrains. In 1983, Chuck Hull, considered the "father of 3D printing," figured out how to print a three-dimensional object by laying a plastic substrate on a platform in layers, heating each layer as it is completed to fix its shape, and then lowering the platform to accept the next layer.
Williams says the difference between 3D printing and 3D bio-printing is the addition of living cells to the material to be printed. The challenge, he adds, has been getting the cells to stay alive during the heating process.
The CII team is using the BAT to build all five parts of the heart (valves, coronary vessels, microcirculation, contractile cells, and the organ’s electrical system) and will eventually assemble them into a bioficial heart. "We’ve made small and large vessels into a construct that is built in such a way that we can, in the future, reconnect that with a patient’s own vascular system,’’ Williams explains. "This is important because we can build these three-dimensional structures in all different shapes and put them into someone, but they have to be hooked up into arteries and veins." The process is painstaking; the past year has been spent on bioprinting small and large vessels into the construct.
Right now, the bioficial large blood vessel is strong enough to hold a suture and the small blood vessel so it can become a functional structure, he says, predicting that within the next two to three years, "some group will make the decision to use 3D printing to build part of [a] child’s heart. It’s always a clinical need that pulls a technology to a patient in the area of cardiac bioprinting."
Williams emphasizes one group alone is not going to be able to achieve 3D printing of organs; it will be accomplished through collaboration. Many other researchers are also studying ways to grow replacement organs and tissues for transplantation. At Carnegie-Mellon University, for example, researchers are also working on bioprinting a heart; since the tissue is a soft material, their approach is to develop a method of printing the organ inside a type of bath that would prevent the material from collapsing on itself.
Researchers at Rice University are trying to develop a transplantation model for engineered tissue that can function like native tissue and give surgeons the ability to implant it like a donor organ, says Dr. Jordan Miller, assistant professor of bioengineering. "We developed a new model of using a 3D printer to fabricate the blood vessels that will connect directly to an artery and get blood flowing during surgery,’’ he explains. The work is detailed in the journal Tissue Engineering Part C: Methods.
Miller’s team previously worked on building 3D structures using molten sugar and living cells, and demonstrating how the fluid flowing through the vessels would keep the cells alive. The current study is looking at whether the structures sustain fluid flow directly from an artery. "We found we could, for three hours,’’ he says. The next study will be to combine the two ideas: taking a tissue made in a lab with cells in it and connecting it directly to an artery to see if the cells will survive in a transplant model.
Whether a 3D organ can someday be constructed is "still an open question,’’ says Miller, "because we as a field don’t fully know all of the structures and functions of all the organs in the body." The shorter-term goal is to build a provisional structure that the cells can survive in and remodel it into something that will function like a native organ.
What makes 3D printing or additive manufacturing so exciting, he says, is the access to every position and coordinate inside a given volume. Because the body’s organs have such a complicated architecture, researchers like Miller believe additive manufacturing will allow for the flexibility and complexity to make structures that look more like native organs.
Like Williams, Miller hopes someday 3D printing can be used to develop custom organ replacements for people, made from their own cells. "We believe this research is on the path to helping with that."
Esther Shein is a freelance technology and business writer based in the Boston area.
Join the Discussion (0)
Become a Member or Sign In to Post a Comment