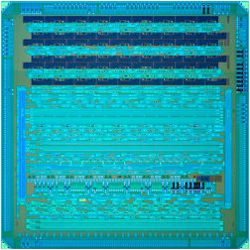
It’s an intriguing idea: a transistor that uses photons of light to perform computations, instead of the electrons used today. “By the end of the decade, supercomputers could be using more light, or ‘photonic,’ components than electronic, and may run at least 100 times faster than today’s generation,” said Alan Huang of Bell Labs in Holmdel, NJ.
However, that quote was found in an Associated Press story from 1990, and the intervening quarter-century has not been kind to that bold projection (for one thing, Bell Labs closed its Holmdel site in 2006). The major reason, of course, is that electronics have continued to improve exponentially according to Moore’s Law, leaving optical transistors and other once-promising alternatives eating their dust. By the year 2000, computer clock rates had indeed increased by nearly a factor of 100, but without any help from optical transistors.
To be sure, optical information handling is a critical staple for communications and the Internet, but using light for computer-scale computation remains a distant dream. Nonetheless, there is a perennial crop of journal articles describing new or improved “optical transistors” using a variety of approaches.
The most mature devices target opportunities in processing optical signals for communications, where optics already provide clear advantages. Yet for computation, the challenge is to find applications where light-based devices could beat conventional electronics at the modest scale that is practical for a nascent technology. Even then, the devices would need critical properties that permit them to be reliably assembled into complex systems. Without a clear view of the entire system that could exploit them, it is difficult to estimate the potential.
Optics versus Electronics
Encoding information in light differs profoundly from doing so using charge. For one thing, electrons exert strong forces on each other, making it easy to manipulate them and to arrange the interactions that enable logical operations. By contrast, photons of light travel long distances without interacting, which makes massive parallelism easier, but means that logically combining two light signals generally requires an intermediary material. Although computing at the speed of light seems impressive, the electromagnetic impulses in ordinary computers propagate essentially as fast.
What actually slows electrical signals is the time needed to charge the wiring capacitance. This charging also stores energy that is usually discarded when the next signal comes along. Because the energy of photons does not depend on how far they travel, they have a power advantage for long-distance connections where the savings overcome the energy costs of generating them in the first place.
In the decades following the invention of the laser in 1960, researchers had high hopes for optical devices that exploited the special advantages of light, such as free-space propagation. Early demonstrations included correlators that scanned an entire image simultaneously for a particular pattern (like a piece of artillery), but these optical analog systems, like their electronic cousins, succumbed to the digital onslaught, with its more robust and flexible design.
Encoding information in light differs profoundly from doing so using charge.
In telecommunications, light transmitted through optical fibers has completely replaced copper wiring for carrying long-distance signals. Telecom providers also regularly amplify and clean up optical signals directly in transit, without converting them to electrical signals. Advanced systems even separate different wavelengths of light and route them to different destinations, for example by sending them through two-dimensional arrays of waveguides built on semiconductor wafers, without the energetically costly optical-electrical-optical conversions.
Communications systems require far fewer optical devices than would be needed for a general-purpose computer. In a 2010 commentary in Nature Photonics, David Miller of Stanford University cautioned that research devices almost never meet all the requirements for robust design and operation of large systems. These include cascadability and fan-out, which ensure the output of a device can provide the input for multiple others, restoration of the signal in both level and quality, and isolation of the input from the output. In addition, most proposed devices are profligate energy consumers.
Still, in recent years researchers have devised many new ways to manipulate light, such as photonic crystals, metamaterials, plasmonics, and even individual molecules. “We would be pessimists indeed not to believe these opportunities will somehow transform information processing,” Miller wrote, “but we will need to be both realistic and creative to get there.”
Light on Light
Even in sophisticated communications systems, electrical control signals determine how the light is manipulated. In the long-sought optical transistor, the control comes instead from a light signal, which the device leverages to modulate a stronger light source that can be likened to an electrical power supply.
One widely used mechanism for modulating light intensity takes advantage of the coherent, well-defined waves emitted by lasers. A beam is first split in two parts that are sent on two different routes; for example, through separate threadlike waveguides on a semiconductor wafer. When they are brought back together, the waves interfere, either enhancing or diminishing their power. Making a small shift in the phase of one of the beams can then turn the output on and off. Optical modulator devices used in telecommunications typically create this shift using electric fields or heat.
Even with this sensitive mechanism, however, the direct effect of a third light beam on the usual waveguide materials is too small to make a practical transistor. Seng-Tiong Ho of Northwestern University in Evanston, IL, and his collaborators devised an optically switched device based on a related two-waveguide device called a directional coupler. They calculate that incorporating a semiconductor material into one waveguide allows another beam to change its gain or loss, and thereby switch a more powerful beam between the waveguides. Ho hopes such devices could allow faster interconnections between, for example, microprocessors. For this application, “it doesn’t have to be very complicated” to be useful, he notes.
Minghao Qi and his colleagues at Purdue University in Lafayette, IN, use a different interference effect that occurs in waveguides that are wrapped into a circle to form a “ring resonator.” If the ring circumference is a multiple of the wavelength, light traveling in a nearby waveguide will be captured in the ring. Heating the waveguide with weak light in a second waveguide can disrupt this delicate match so the original light instead passes by freely. The device, which can be made with standard silicon-processing techniques, shows optical gain and isolation of the input from the output, both critical for larger circuits. Such optically gated devices might function in spite of electromagnetic interference, Qi suggests, or they could be used in artificial neural networks. “It’s important for us to understand what technology potentially has any niche applications that may allow it to survive and then it can grow.”
One possible niche is extending communications, where optics already beat electronics. Research by semiconductor giants like IBM and Intel, as well as projects funded by the U.S. Defense Advanced Research Projects Agency (DARPA), has demonstrated compact, high-speed optical devices directly integrated with silicon electronics. So far, electrical interconnections still dominate, not just within chips, but even between chips on a board. Nonetheless, optical communications are appearing in some advanced systems for interconnecting boards, and are likely to displace electronics at ever-shorter distance scales.
To provide new options for merging optics and electronics, Swastik Kar of Northeastern University in Boston, MA, teamed up with nanotube expert Young Joon Jung to build optically switched devices on a semiconductor wafer. Although the output of these devices was electrical current, not light, the team demonstrated logical operations combining light and electrical inputs, including a two-bit adder and a four-bit analog-to-digital converter. “We believe that this kind of device,” Kar says, “will allow a better merging of the processing and transfer of information.” An important feature of the nanotube device is that the current in the off state is reduced by a factor of 105, much more than for typical photodiodes. Such small off-currents are critical when large numbers of devices are used on a chip.
Seeking a New Niche
Some researchers see an opportunity for optics in a technology that does not yet exist: quantum computing using quantum bits (qubits) that exist in multiple states simultaneously. “If you had 100 qubits, you could be competitive or better than current classical computers for certain types of calculations,” says Vladan Vuletić of the Massachusetts Institute of Technology in Cambridge, MA. He stressed, “You don’t need enormous numbers.”
Vuletić and his colleagues have made the ultimate optical transistor, in which a light beam is turned on and off by the absorption of a single photon. To do this, they placed a cloud of about 20,000 cesium atoms in an optical cavity and cooled them to a few millionths of a degree above absolute zero. Because the light bounces back and forth hundreds of thousands of times, the absorption of a single photon of a second beam by one of the atoms changes the resonance enough to turn the beam on and off.
Of course, this apparatus is not very practical, even for a dedicated supercomputer. “To really make multiple quantum gates to do even some simple calculations with photons, probably these systems that we use now are too bulky,” Vuletic acknowledges. He and others have recently demonstrated much smaller resonators could be assembled together over the next five years or so.
Still, it will be years, if ever, before any type of quantum computing becomes established, and even then other non-optical schemes may be used for implementing the qubits. So for now, computing with light is still waiting for its time to shine.
Further Reading
Krishnamurthy, V., Chen, U., Seng-Tiong Ho
“Photonic transistor design principles for switching gain >= 2,” Journal of Lightwave Technology 31:2086 (2013).
Varghese, L., Fan, L., Wang, J., Gan, F., Niu, B., Xuan, Y., Weiner, A., Qi, M.
“A Silicon Optical Transistor,” http://arxiv.org/abs/1204.5515
Kim, Y., Jung, H., Park, S., Li, B., Liu, F., Hao, J., Kwon, Y., Jung, Y., Kar, S.
“Voltage-switchable photocurrents in single-walled carbon nanotube–silicon junctions for analog and digital optoelectronics,” Nature Photonics 8, 239 (2014)
Chen, W., Beck, K., Bücker, R., Gullans, M., Lukin, M., Tanji-Suzuki, H., Vuletic, V.
“All-Optical Switch and Transistor Gated by One Stored Photon,” Science 341, 768 (2013).
Join the Discussion (0)
Become a Member or Sign In to Post a Comment