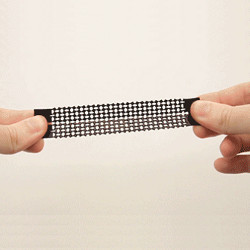
When you picture a robot, you likely envision one large and rigid, with limited movement and an outer shell that is hard to the touch. Several projects currently underway seek to change that, with the use of soft, more human-like artificial skin.
Artificial skins include any surface-based device or distributed network of sensors that enable an agent to perceive mechanical deformations, touch, temperature, vibration, and/or pain, according to Ryan Truby, a post-doctoral fellow in the Massachusetts Institute of Technology (MIT) Computer Science & Artificial Intelligence Lab (CSAIL). Engineers are working to create skins that include as many of these sensations as possible, while also possessing high sensitivity and spatial resolution in sensing, he adds.
“Artificial skin will liberate today’s robots and bring them to a higher level of consciousness,” notes Yiannis Aloimonos, a professor at the University of Maryland who leads the school’s Computer Vision Laboratory (CVL). “They will become much safer, especially when operating near people.”
Robotic skin cells, in one sense, can do more than animal skin cells because they can sense proximity to a surface for anticipating and avoiding accidents, Aloimonos says. “Having this additional sense, the robots will be able to perceive their environment in greater detail.”
The fusion of vision and tactility will make possible many new tasks that were not possible before, Aloimonos says. One problem being studied in his lab is how to teach robots to use tools. When humans use tools, we remember the feeling of a tool in our hands, and use this information to learn how to control its movement. “Recognition from vision and touch also becomes a new powerful technique,” he says. “In general, any tasks where the robot comes into contact with something can be done better.”
Figure. University of Texas at Arlington’s patented smart skin technology, which the university says will give robots more sensitive tactile feeling than humans.
Robots today are not able to help in dire situations like natural disasters. “There is a conspicuous absence of robots whenever we are in trouble,” including in the midst of the coronavirus pandemic, says Aloimonos.
That is not to say the work robots do has not been important; it is just limited. Up until now, industrial and commercial applications of robotics have been large in scale and focused on factories, “and they are incredibly well-engineered for what they need to do, but they are not designed for use with humans,” says Carmel Majidi, director of the Soft Machines Lab and an associate professor of mechanical engineering at Carnegie Mellon University (CMU).
One of the main ideas for developing soft robotics is to create machines that are safe for physical interaction with humans and won’t injure us in a collision, Majidi says.
Often, industrial robots must be caged behind metal fences to prevent them from harming humans who unwittingly enter their areas of operation, says Majidi, who is spearheading an effort to develop new classes of soft materials in the fields of soft matter engineering and soft robotics.
A significant step in making machines safe to engage in physical contact with humans is to build them from materials that share lot of the same properties as us: water, fluids, soft materials, and nervous tissues that are soft and stretchy, Majidi says.
“Tactile sensing can be thought of as an ‘artificial skin’ technology that enables robots to have a sense of touch,” he says. “They are used in a wide range of robotics applications, from humanoid robots to wearable technologies. They can also be used in soft robotics, but this is not their primary goal.”
Autonomy in robotics can be advanced with improved perception, Truby says. “The abilities to sense touch, stretch, temperature, vibration, and many other forms of tactile and proprioceptive feedback are critical for the autonomy of any robot tasked with operating in the real world.”
Artificial robot skins are a straightforward way to begin introducing these perceptual capabilities in robots, says Truby. “Innovations in robotic skins will likely be key to advancing the autonomy of robots designed for real-world deployment.”
Additionally, he says, softer skins will improve the safety of human-robot interactions and also open up new ways for us to interact with machines.
These advances would take humans out of harm’s way, he says. The field of soft robotics has generally shown that soft materials like the rubbers and hydrogels soft robots are being constructed from “do not just make robots safer for human-robot interactions; they also offer important advantages over materials like metals and thermoplastics that are used in ‘rigid’ robots today,” Truby says.
These advantages can help soft robots become more resilient in harsh environments such as in a forest fire, for example, compared to their rigid counterparts, he says. “Thermoplastics would melt or burn from the heat of fires. “Excellent thermal conductors like metals can reach high temperatures in a fire, potentially damaging other onboard components due to heating. In contrast, soft robotic materials avoid these issues because they are often good thermal insulators and can demonstrate higher flame resistance.”
Truby and his colleagues at MIT developed a system of soft sensors that cover a robot’s body to enable its awareness of motion and position. “If we want to have soft-skin robots in the real world, we need to bring skins and sensing capabilities to these systems that can provide the feedback needed to autonomously control the robot’s motion,” Truby says. “We are going to have to solve the material engineering challenge of bringing those capabilities into soft robot bodies.”
The research team tested its system on a soft robotic arm equipped with very simple sensors to predict its own position as it autonomously swings. The test proved eye-opening: “The very simple, inexpensive sensors we gave this soft robot arm enabled a deep neural network to provide a satisfactory estimate of the arm’s 3D configuration as it moved about,” Truby says. “To our knowledge, this is a first for the field of soft robotics.”
He says the team is always working to improve its soft robots’ capabilities and is now focused on improving the accuracy of the configuration estimations through new sensor skin designs and deep learning methods. “We are also working to use this feedback to dynamically control the arm for a variety of applications.”
“We are working to improve the materials that go into these skins to enable tactile sensing, as we would expect from a sophisticated soft robot skin.”
There are many types of artificial skins, but many are expensive to make, not easy to interface with soft robots, and are not scalable, Truby says. “We’re fighting against cost and scalability,” and accessibility. “The advantage of soft robots is they are cheap.”
The MIT researchers worked with “commodity conductive silicone rubbers available right off the shelf,” he says. Comparable skins comprised of other types of conductive rubbers, or materials like liquid metals or ionically conductive gels, have been made through relatively simple and inexpensive techniques such as 3D printing and molding, he says.
Ordinary rubbers is a good insulator but not a good conductor, Truby says. The materials used by the researchers “have conductive particles in them, so we can conduct electricity and can create sensory skins from them.”
More complicated skins that can perceive multiple sensations and/or possess a higher density of “receptors” for these stimuli are often made with slower, more expensive microfabrication techniques, Truby says. “While micro-fabrication methods open up opportunities for more readily building with materials like thin metal films and conductive polymers, it’s often very expensive to scale these techniques to produce artificial skins of large area,” he says. “Thus, when designing artificial skins, there is a clear trade-off between performance, size or area, and cost.”
Yet more expensive artificial skins can provide “a very high resolution of tactile and touch feedback,” he notes. They provide a lot more functionality and are commonly used in prosthetics, Truby says. “But these available methods are expensive to implement, do not scale well for large soft robots like ours, and can be difficult to interface with soft robots,” he says. “Our approach represents a new strategy for rapidly, inexpensively, and easily giving soft robots important perception capabilities, which remains a challenge in soft robotics.”
Right now, MIT’s soft robotic skins are not appropriate for tactile or touch sensing, Truby says. “We are working to improve the materials that go into these skins to enable tactile sensing, as we would expect from a sophisticated soft robot skin. We’re also working on algorithms that complement these simple soft sensor skins and enable new contact sensing motifs from external cameras and actuation inputs.”
Majidi’s group at CMU is researching materials and systems that combine mechanical, electrical, and thermal properties and can function as artificial skin, nervous tissue, and muscle for soft robotics and wearables.
His lab created a self-healing material that repairs itself under extreme mechanical damage, according to CMU. The soft-matter composite material is composed of liquid metal droplets suspended in a soft elastomer; when damaged, the droplets rupture to form new connections with neighboring droplets and reroute electrical signals without interruption. Circuits produced with conductive traces of this material remain fully and continuously operational even when severed, punctured, or with material removed. The goal is for electrical connections to spontaneously restore themselves when damaged, Majidi says.
Applications for the soft material include bio-inspired robotics, human-machine interaction, and wearable computing. Because the material also exhibits high electrical conductivity that does not change when stretched, it could be used in power and data transmission, according to CMU.
The greatest challenge is delivering adequate power to electronics within these skins, Majidi says. Skin that is soft and stretchable cannot be charged with a “big hulking battery … so there’s the challenge of creating batteries that are soft and flexible,” which is one project his lab is working on.
The amount of energy contained in a small lithium battery is limited, he says, and if you want to incorporate sensors and electronics in artificial skin, today they are not capable of detecting their environment or physiology. Current lithium battery cells have enough power to operate the sensors that detect the environment or physiology, he says. “However, they don’t have enough power to also operate the microprocessor used to process the signals and the radio transceiver used to transmit the signals wirelessly. For there to be enough power for long-term sensing, signal processing, and wireless data transmission, the current miniature battery would have to be replaced with a much larger battery.”
A larger battery would add to the rigidity of the soft artificial skin technology, Majidi says. This introduces a challenge with supplying power to an artificial skin.
There are two potential solutions. The first is to use a battery that is soft, flexible, and stretchable, he says. This type of battery could be larger in size than the existing miniature lithium batteries and therefore supply more power, Majidi says. Moreover, because it is soft and compliant, it will not add to the rigidity of the soft artificial skin.
Another solution is to use energy-harvesting to convert energy sources in the environment into electricity. “My lab is currently working on harvesting energy from body heat using soft and stretchable thermoelectric generators, as well as from knee and elbow motions using a soft conductive material for triboelectric energy harvesting.”
Eventually, if enough energy can be harvested, “It could power motors to get robots to move and actuate,” he says. “That’s the really critical step.”
The inability of artificial skin to sweat is another challenge. Soft robots produce heat, and it is important to have materials that can manage that heat, Majidi says. “So we’ve been creating soft thermally conductive rubber that can help manage or dissipate the heat that’s generated by these machines and electronics.”
“We’ve been creating soft thermally conductive rubber that can help manage or dissipate the heat that’s generated by these machines and electronics.”
Sweating and synthetic skin are also being studied at Cornell University, where scientists have created a soft robotic muscle that stays cool and mimics how a hand moves.
The ability for a muscle to stretch is helpful in adapting to unpredictable circumstances, like walking through a disaster zone with rubble everywhere, says Cornell associate professor Rob Shepherd. “It’s hard to predict what the next meter of terrain will look like.”
Shepherd and other Cornell engineering professors use polymers—in this case, rubber—for the skin, since it stretches and is similar to human skin, he says. Like Truby, he adds, “It is also pretty cheap, and you can make them self-heal.”
Such benefits come at many costs, Shepherd notes, one being that polymers and rubber don’t conduct heat very well, unlike metal. “Cooling robots hasn’t been as much of a problem as it will be in future for these polymer ones,” he says. “If we expect them to do a lot of work quickly, they’ll generate heat and that will damage the robot, so we have to dissipate the heat somehow.”
The Cornell researchers developed a system that automatically dilates pores in an actuator when the temperature reaches 86 degrees Fahrenheit. The idea is when the pores dilate, it allows for an increase in the rate of evaporation and cools the muscle’s body, similar to perspiration in hot-blooded animals, according to Anand Kumar Mishra, a post-doctoral student at Cornell working on the project. The job of the fluids is to actuate the muscle, says Mishra, “But when the robotic body temperature elevates, then the actuating fluid can also be used for perspiration to regulate the body temperature.”
This will enable a robot to operate for long periods without overheating, Shepherd said.
The idea of an artificial skin for robots has been researched for decades, and there are numerous applications being developed with human-robot collaboration (HRC), says Werner Kraus, head of the Robot and Assistive Systems department at the Fraunhofer Institute for Manufacturing Engineering and Automation IPA, in Stuttgart, Germany. “Artificial skin could enable this type of [HRC] interaction, since its sensors can detect a contact between human and robot and stop the robot’s movement according to safety requirements.”
Artificial skin offers more sensor data to perceive the environment, Kraus says. “In times of powerful machine learning algorithms, this data can be the enabler for implementing software on robots that makes them more autonomous and more efficient.”
Liu, D., Su, L., Liao, J., Reeja-Jayan, B., and Majidi, C. “Rechargeable Soft-Matter EGaln-Mn 02 Battery for Stretchable Electronics,” Advanced Energy Materials, Wiley Online Library, https://onlinelibrary.wiley.com/doi/abs/10.1002/aenm.201902798
Carmel Majidi: Self-Healing Electrical Material, https://www.youtube.com/watch?v=N_ijvkl51LM
inSPIREd – Dr. Ryan Truby – Can soft materials revolutionize the meaning of robotics?, https://www.youtube.com/watch?v=eRTyWgTHQZI
Chortos, A., Liu, J., and Bao, Z. “Pursuing prosthetic electronic skin,” Nature Materials, https://www.nature.com/articles/nmat4671
Join the Discussion (0)
Become a Member or Sign In to Post a Comment