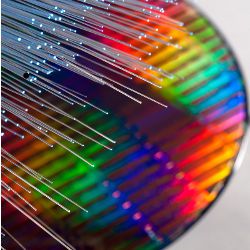
Although photons of light dominate the long-distance information superhighways of the Internet, electrons remain the workhorses that move and process information at the local level. Visionaries have imagined for decades that power-efficient light-based communication would migrate to ever-shorter distances and eventually move onto silicon chips alongside transistors at the centimeter scale.
After more than a decade of research and development, chips with onboard photonics are poised to enter the marketplace. Rather than connecting nearby electronic circuits, however, these devices aim to provide high-bandwidth connections over hundreds of meters or longer. Some devices could provide customized solutions for servers and exascale computing, whereas others target the large but cost-sensitive data center market.
To address these applications, technologists have adapted and repurposed the latest processes for fabricating complementary metal oxide semiconductor (CMOS) chips to create optical components. Together with novel packaging schemes to connect chips with optical fibers and standards to ensure interoperability, these silicon-photonics chips could at last bring light and silicon into intimate contact. If this framework is commercially successful, it could also open the door for realistic consideration of other ideas for chip-based optics of which researchers have long dreamed.
Putting the Pieces Together
Optical communications systems require a broad palette of components. These include lasers to supply light of various colors (wavelengths), modulators to imprint signals on them, devices to combine and separate wavelengths, waveguides to route light, and detectors to extract the signal. Processes have been devised to build all of these—except the light source—using standard silicon processing techniques.
Probably the easiest components to make are waveguides that precisely route light signals on a chip. These can be made by creating a rectangular wire of semiconducting silicon, which is transparent to infrared light but has a high index of refraction that traps the light, and surrounding it with silicon dioxide insulator. Silicon-on-insulator processes, which are now widely available for CMOS, can easily create a low-loss waveguide by patterning the same semiconductor layer used for transistors and then covering it with insulator.
Another device that has benefited from recent innovations in CMOS processing is the light detector. Alloys of silicon and germanium are now used by many manufacturers, so these materials can now be used without risk to transistors. The alloy composition can be chosen to absorb the signal light that passes freely through silicon, liberating electrons that can be detected electrically.
Because any modifications to the CMOS process pose a risk to transistors, it is difficult to develop a credible optical functionality on chips without access to the process line. William Green, from IBM’s Research Center in Yorktown Heights, NY, says he and his colleagues worked closely with engineers at their Burlington, VT, manufacturing facility to implement their optical devices.
The resulting process is now formalized in a kit that lets external clients design optical components, along with analog and digital electronic circuitry. “It’s almost transparent to the designer that there are optical components,” Green says. IBM divested its foundry operations to GlobalFoundries in July 2015; Green’s team still works with the Burlington team and its clients.
IBM developed an approach in which mechanical structures are added to completed lasers to let them self-align to the silicon phototonics with sub-micron accuracy.
The IBM process includes detectors incorporating pure germanium for operation in the 1.3-micron wavelength range that is specified in interconnection standards. It also supports sophisticated devices that use interference effects to separate slightly different wavelengths of light. Sending multiple signal beams down the same fiber, or wavelength-division multiplexing, allows for a very high bandwidth interconnection with electronics that only has to operate at a fraction of the speed. For example, last year the IBM team showcased their technology with a 100Gb/s transceiver reference design that combined four wavelengths, each carrying 25Gb/s.
Light Sources
For detectors, the intrinsically weak optical coupling of silicon and germanium to light can be overcome using waveguide-based designs. For light sources, however, these group-IV semiconductors are at a serious disadvantage relative to traditional laser materials based on III-V semiconductors such as GaAs, InP, and their relatives.
Direct growth of III-V materials during silicon processing is difficult for a variety of reasons. In one alternative approach, researchers demonstrated laser action from silicon itself under some conditions. However, the most mature strategies use hybrid integration, in which III-V materials are grown in separately optimized systems and then brought together with the silicon.
Intel, for example, has described a hybrid silicon laser in which a stack of III-V materials is bonded to a silicon wafer containing a predefined waveguide. The resulting sandwich is then processed to create a laser with a selected wavelength, whose output is tightly coupled to the waveguide. Intel had promised 100Gb/s silicon photonics chips in 2015, but the planned release was pushed into 2016 because of unspecified production problems. Intel declined to comment for this story.
IBM has developed a different approach, Green says, in which mechanical structures are added to completed lasers to let them self-align to the silicon photonics with sub-micron accuracy. These efforts highlight the critical need for low-cost, precise packaging for optical signals if silicon photonics is to reach its potential.
Zero-Change Process
An alternative to intimately bonding a laser to the silicon chip is to supply its light using a fiber from above, using a structure on the chip to direct it laterally into a waveguide. “We want to treat the lasers like any other power supply in the system,” says Vladimir Stojanović of the University of California, Berkeley. Companies like Luxtera, which is another major player in silicon photonics, have refined active alignment techniques for this sort of packaging, he says.
Stojanović and his collaborators at Berkeley, the University of Colorado, and the Massachusetts Institute of Technology are championing a “zero-change” process that constructs optical devices using an unmodified CMOS flow. In a long-running project supported by the U.S. Defense Advanced Research Projects Agency (DARPA), they are aiming to use optical connections to relieve the information bottleneck between processors and memory. In December, they published a demonstration of a 2.5Gb/s optical link between a full processor and 1MB memory on separate chips, each fabricated alongside the optics using IBM’s SOI foundry process.
This demonstration used only a single wavelength of about 1.18μm, which is detected by the silicon-germanium alloy used in the CMOS process. The design, however, could modulate multiple beams in a single waveguide, through a series of micro-ring modulators about 10 microns in diameter, which can be tuned to different wavelengths. The downside of these devices is they are very sensitive to process variations as well as temperature, so the team included an active circuit to lock them to the desired wavelength. This effort was important, Stojanović, says, because stability is “the most critical thing that stands in the way of people adopting rings more broadly.”
Indeed, most silicon-photonics projects merge multiple beams after encoding them with data using single-pass devices called Mach-Zender modulators, which are more stable but generally more than order of magnitude larger. For the transceiver market, though, IBM’s Green says the area of the silicon is not a big part of the cost relative to packaging and testing.
On-Chip Optical Memory
One function that could be useful for optical chips is direct storage of optical information. Last year, Harish Bhaskaran of the U.K.’s Oxford University and Wolfram Pernice of the Karlsruhe Institute of Technology and the University of Muenster in Germany, with their colleagues demonstrated a non-volatile memory on a silicon wafer that is written and read optically.
The team exploited an alloy of germanium, antimony, and tellurium, denoted GST, that stably but reversibly switches between a crystalline atomic arrangement and a disordered, amorphous phase. Such phase-change materials have been investigated for electrical memories because the two phases differ in resistivity. Moreover, GST is used in commercial rewriteable optical storage discs, exploiting differences in the reflection of visible light. The new scheme instead exploits the difference in the absorption of light with the 1.5-micron wavelength used for telecommunications.
The team placed a GST film on top of a rectangular waveguide on a wafer, close enough to suck out light energy traveling along the waveguide.
A high-power pulse that melts the GST, when followed by fast cooling, produces an amorphous film. Gentler heating returns the GST to the crystalline state. In the demonstration device, the larger absorption of the crystalline state reduces the light transmitted down the waveguide by about 20%, a difference the researchers can easily detect in the lab. In fact, they say their measurement can discriminate many degrees of crystallization, allowing the storage of multiple bits, because the absorption level drifts less over time than the corresponding resistivity, even when the reading beam is present. Multiple bits would allow more compact optical storage, although probably still much larger than electronic memory cells. Bhaskaran suggests the scheme could help relieve the communication bottleneck and might be particularly useful in future designs based on neuromorphic principles.
Although the kind of on-chip communication these memories might enable could be important someday, for now “the need is really pressing on chip to chip,” Stojanović says. If on-chip optics becomes widespread for inter-chip applications, “we could make things easier for the optics to conquer that last millimeter.”
Further Reading
Bainbridge, L.
Ironies of automation. New Technology and Human Error, J. Rasmussen, K. Duncan, J. Leplat (Eds.). Wiley, Chichester, U.K., 1987, 271–283.
Monroe, D.,
Still seeking the optical transistor, Communications, October 2014.
Monroe, D.,
Neuromorphic Computing Gets Ready for the (Really) Big Time, Communications, June 2014
D.M. Gill et al.,
Demonstration of Error Free Operation Up To 32 Gb/s From a CMOS Integrated Monolithic Nano-Photonic Transmitter, IEEE J. Quant. Elect. 21
C. Sun et al.,
Single-chip microprocessor communicating directly using light, Nature 528
Join the Discussion (0)
Become a Member or Sign In to Post a Comment