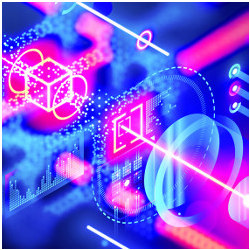
The march toward functional quantum computing devices has taken a long and winding road. Although the concept has been around since the late 1970s, when physicists Paul Benioff, Richard Feynman, and others began to explore quantum information theory, only recently have actual devices begun to take shape. Several companies, including IBM, have developed prototype quantum computing systems, while many research organizations have experimental devices in early stages of development.
Yet unlike classical computing, which has evolved over more than 70 years and is now mature, quantum computing, which harnesses quantum physics to leverage the uncertainty of a quantum state versus the certitude of a classical state, remains largely uncharted territory. An enormous amount of research is currently focused on ways to create or utilize quantum bits (“qubits”) to construct quantum mechanical systems that can harness physical events in nature to solve complex computing problems lying outside the practical grasp of classical systems. At the moment, qubit research remains in a relatively nascent state and, as a result, it is not clear which approaches will ultimately prevail.
“There’s an enormous push to develop different quantum systems that could prove stable enough to be useful,” says Michael Cuthbert, director of the National Quantum Computing Center in Oxfordshire, U.K.
For now, qubit research is heavily focused on a handful of key technologies: superconducting circuits, trapped ions, photonics, ultra-cold atoms, spins in silicon, color spins in diamonds, and an emerging area known as topological insulators.
Key questions and challenges remain, including how to scale devices while reducing noise and errors to the point where qubit devices become useful. Says Travis Humble, deputy director of the U.S. Department of Energy (DOE) Quantum Science Center at Oak Ridge National Laboratory, “We are reaching a critical point where quantum computing technology is advancing rapidly. Over the next few years, we may see actual devices emerge that solve real-world challenges in drug development, financial modeling, cyber-security, and physics.”
A Quantum Challenge
Quantum computing devices are emerging in several shapes and forms—and they use radically different methods to process calculations. The common denominator is that all these systems use a quantum circuit to handle calculations. As with bits in classical computing models, qubits operate in a 1 or 0 state. Unlike classical computing, the information is challenging to record; moreover, the physics of the quantum realm unlocks the possibility of the occurrence of both 1s and 0s together. As a result, it is necessary to measure the qubit before and after a change, to understand its state at any precise moment.
Qubits typically maintain useful information for very brief periods of time, in some cases no more than a millisecond, as they are prone to high levels of noise and errors. By comparison, silicon can run a billion classical operations per second for a billion years before a statistical error occurs. “It’s possible to mitigate this instability through error correction,” Cuthbert says, “but the error correction comes with significant overhead. It’s necessary to reach somewhere around 1,000 physical qubits to get to the point where the error correction works effectively, creating a single logical or ‘forever’ qubit.”
So far, researchers and engineers have been able to build qubit devices to a scale of just over 60 qubits. What is needed are devices that can reach at least 1,000 qubits, or for a fully fault-tolerant error-corrected machine, 1 million qubits, experts say. Although better algorithms can address part of the noise and error problem by calculating more efficiently, they cannot address it entirely.
The goal, then, is to build qubit devices that break through today’s barriers and serve as components for quantum computers. While digital devices encode electrical signals in a string of ones and zeros, quantum systems require a highly stable two-level system that can establish a superposition of a one or zero, as well as the entanglement of states between different qubits.
Despite all the gains, the path to quantum computers remains lined with obstacles.
This two-level system must bring quantum bits sufficiently close together to couple, in order for the device to generate usable output. In addition, a quantum computing device requires circuits that can detect and encode quantum states and generate data that can be used to understand events. The takeaway? “There are many different potential directions for quantum technology to take,” says Arun Persaud, a staff scientist at the Department of Energy’s Lawrence Berkeley National Laboratory.
Qubit Devices Emerge
In 2019, scientists at Google announced they had passed a critical threshold in quantum computing: they managed to build a 53-qubit device that could solve in about 200 seconds a problem that would require upward of 10,000 years on the Summit supercomputer at Oak Ridge National Laboratory to complete. Although some in the computing community disputed the results, and it was widely acknowledged that the experiment did not solve any meaningful computing problem, many saw it as proof that quantum devices delivered what Google researchers then labeled “quantum supremacy.”
Google’s method relied on transmission line shunted plasma oscillation qubits, generally known as transmons, to calculate the problem. Transmons are a type of superconducting charge qubit that displays reduced sensitivity to charge noise. The same technique, which requires chilling superconducting metal to near absolute zero, has been used by other commercial vendors to produce quantum computers. A transmon manipulates ratios between different energy levels inside of the superconducting device, and scaling up a system of these devices requires sophisticated engineering. “While today’s devices are relatively primitive in terms of the capacity and the number of instructions they can perform, there’s been remarkable progress with the technology over the last five years,” Humble says.
Another type of qubit device involves individual ions trapped in a stable configuration. These charged particles may consist of calcium, phosphorous, or other elemental atoms that are injected as a dilute gas into a vacuum system. An electrical or optical system, such as a laser, is used to induce coupling between the atomic quantum states. The technique has attracted the attention of several organizations, including Google and Honeywell, though these devices also remain in the tens of qubits, rather than the hundreds or thousands expected for commercial applications.
A third major area of research involves photonic systems. Instead of using electrons to store and carry information, these devices record information in the quantum state of light known as photons. The advantage of this approach is that researchers are familiar with the laser, which is itself a quantum technology. The challenge with the technique is getting photons to interact so the system can process the needed information. However, “It’s a very promising area because of the speed at which photons travel,” Humble says. “You can use them to distribute information between locations.”
Meanwhile, researchers continue to explore other types of qubit devices. For example, one early-stage technology uses topological insulators to encode information that is intrinsically resilient to the noise. The interior of these material systems functions as an insulator, while the surface contains conducting states that allow electrons to move easily. The exotic quantum states that arise are then used in a form of computation called ‘braiding’.
Like weaves in a fabric, braiding exchanges the relative ordering of the electrons. However, due to their quantum nature, they perform meaningful calculations. Microsoft, which has partnered with the Quantum Science Center at Oak Ridge, has a leading commercial stake in the development of a topological quantum computer using ultra-narrow nanowire devices, and the laboratory is exploring new materials systems that could demonstrate these effects.
Qubit by Qubit
The field of quantum computing took an important step forward in February, when Persaud and a team at Berkeley National Laboratory discovered a possible way to form self-aligned color centers that are close enough together to potentially push scalability to upward of 10,000 qubits—about two orders of magnitude greater than other ion-trapping technology.a The group accomplished this feat by using ion beams to generate artificial color centers in diamonds; it now is working on creating samples with single lines of color centers and measuring their quantum behavior. The color centers are microscopic defects that involve a nitrogen atom residing next to an empty space in the structure of the crystal.
By exciting passing ions to form nitrogen-vacancy centers in the diamond lattice, the centers and adjacent carbon atoms can serve as solid-state qubits. What’s more, the crystal lattice helps protect their coherence and keep them entangled. This has significant ramifications because the quantum system can store data without requiring a cryogenic environment. “You can use optical detection of photons, a well-established technology, to detect and measure the state of qubits,” Persaud says.
Also, in May of this year, a team at Washington University in St. Louis led by Jung-Tsung Shen, an associate professor in the Preston M. Green Department of Electrical & Systems Engineering of the university’s McKelvey school of Engineering, developed a high-fidelity two-bit quantum logic gate based on light.b It bumps up efficiency by an order of magnitude using photonic dimmers, a type of photonic interaction that exists in both space and frequency. When a single photon enters a gate, there is no reaction; however, when two enter simultaneously, it is possible to perform two-bit operations within a quantum framework.
Still another key breakthrough took place in June, when researchers at the Massachusetts Institute of Technology (MIT) found a way to reduce errors in two-qubit gates through fidelity advances in tunable couplers—systems that allow researchers to switch on and off various operations while preserving qubits.c In order to mitigate qubit-to-qubit interactions that lead to errors, researchers tapped higher energy levels within the coupler to cancel out problematic interactions, and ultimately, errors. The method “helps address one of the most critical quantum hardware issues today,” says Youngkyu Sung, an MIT graduate student in electrical engineering and computer science.
The Path Ahead
Despite all the gains, the path to quantum computers remains lined with obstacles.
Because decoherence takes place so rapidly, better material purity and lower noise in electronic systems are critical, says Erik DeBenedictis, co-chair of IEEE Quantum, an initiative that serves as IEEE’s leading community for all the organization’s quantum technology projects and activities. Many current systems simply produce too much heat, DeBenedictis says; as engineers attempt to scale quantum devices, “There are too many qubits pushed too close together and there’s simply too much crosstalk.”
Conquering the scaling problem will not be easy. Says Cuthbert, “Right now, the challenge is to produce an ensemble that’s large enough and displays sufficient noise mitigation to provide useful results. We also need to develop better algorithms for use cases that demonstrate a clear quantum advantage.”
Finally, there is a need to better understand different behaviors that take place on various quantum platforms, Cuthbert says. “Some are noisier than others. The task isn’t only to discover the perfect qubit and couple it with the next perfect qubit. There are issues involving connectivity between qubits and the speed at which those interactions take place.” Adding to the challenge: different types of qubit devices and technologies have varying levels of tolerance to noise, something that may require different methods of error mitigation, correction, and control.
Nevertheless, the race to develop functional quantum computers continues. Over the next few years, qubit devices are likely to mature, scale, and evolve into far more advanced systems. For example, IBM has promised a 1,000-qubit machine by 2023.d
Says Persaud, “Quantum computing is a big field and there are many aspects to it. Right now, there’s no consensus on which approach will win out. We may see one approach ultimately prevail, but we also might also see different types of systems for different quantum computing uses.”
Sung, Y., Ding, L., Braumüller, J., Vepsäläinen, A., Kannan, B., et al.
Realization of High-Fidelity CZ and ZZ-Free iSWAP Gates with a Tunable Coupler, Physics Review, Vol. 11, issue 2. June 16, 2021; https://journals.aps.org/prx/abstract/10.1103/PhysRevX.11.021058
Lake, R.E., Persaud, A., Christian, C., Barnard, E.S., Chan, E.M., et al.
Direct Formation of Nitrogen-Vacancy Centers in Nitrogen Doped Diamond Along the Trajectories of Swift Heavy Ions. Applied Physics, Volume 118, Issue 8. February 24, 2021; https://aip.scitation.org/doi/10.1063/5.0036643
Chen, Z., Zhou, Y., Shen, J., Ku, P., and Steel, D.
Two-photon Controlled-Phase Gates Enabled by Photonic Dimers, Physics Review A, Volume 103, Issue 5. May 2021; https://journals.aps.org/pra/abstract/10.1103/PhysRevA.103.052610
Hays, M., Fatemi, V., Bouman, D., Diamond, C.S., Serniak, K., et al
Coherent Manipulation of an Andreev Spin Qubit, Cornell University, January 17, 2021. https://arxiv.org/abs/2101.06701
Join the Discussion (0)
Become a Member or Sign In to Post a Comment