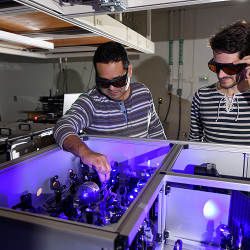
Organic semiconductors, such as those used in inexpensive solar cells, have the advantage of sidestepping the need for the expensive chemical vapor deposition (CVD) process used to create conventional silicon solar cells. CVD requires the use of ovens heated to thousands of degrees, in which vaporized silicon settles onto wafers to grow perfect stacks of single-crystal atomically thin layers inside the oven. Now not just pure organic, but hybrid organic-inorganic solar cells made from perovskites, can also be dissolved into liquids which can be inexpensively painted onto wafers at room temperature.
Researchers at the Georgia Institute of Technology (Georgia Tech), the Center for Nano Science and Technology at PoliMi—Istituto Italiano di Tecnologia, Milan, Italy, and the Université de Mons in Belgium, recently expanded the range of applications for hybrid organic-inorganic perovskite (HOIP) semiconductors by explaining their underlying quantum mechanisms, thus unlocking their potential to emit light as LEDs, lasers, and even room-temperature quantum gates.
"The material itself is not where our innovations are found," said Georgia Tech professor Carlos Silva. "Rather, our contribution is in the understanding of how many complex interactions come together to define the optical properties of perovskites. Three-dimensional bulk perovskite solar cell materials are already here, but our materials are 2D (two-dimensional) films that are better suited for LEDs and lasers, as well as for quantum condensation phenomena including the possibility of room-temperature quantum gates."
Perovskite solar cells have one big disadvantage—in their bulk form, they are very sensitive to oxygen and humidity, which quickly degrade their performance. This is solved by encasing them in glass or special polymers, but when deposited as 2D crystals, they are much less sensitive to the environment, according to Silva. By taming the complex 2D deposition process, the researchers hope to use both subtractive and additive deposition to create a new family of advanced semiconductor devices.
"These materials are processed in solution like in perovskite solar cells, but the 2D crystal formation process is more complex," said Silva. "But one can indeed use subtractive lithographic techniques post deposition. Others are experimenting with ink-jet printer additive deposition."
Hybrid organic-inorganic perovskite semiconductors are suited for many electro-optical applications, according to Silva, using quantum confinement in optical micro-cavities to energize their activity. The crystalline structure of a perovskite is very complex, using one large and one small cation (a negative ion with more electrons than protons) bonded to an anion (a positive ion with more protons than electrons) surrounded by an octahedron of anions. The result is similar to the structure of some novel superconductors. The complexity of its flexible crystalline lattice is also more energetic than that of typical organic semiconductors.
"Many nonconventional superconductors and topological insulators have a perovskite structure. Here, we consider 2D derivatives of a particular perovskite that is a semiconductor, in which single-lattice planes are separated and confined from each other by long organic molecules," said Silva. "The electrons that give rise to the semiconductor properties are confined to a 2D plane involving single-layer lead-halides—much like a semiconductor quantum well, and also akin to other 2D materials such as transition metal dichalcogenides like molybdenum disulfide [MoS2]."
The particular perovskite synthesized by Georgia Tech's collaborator on the project, researcher Ajay Ram Srimath Kandada at Istituto Italiano di Tecnologia (currently in residence at Georgia Tech), can be considered a wide-band semiconductor whose electron mobilities are lower than those of the best semiconductors today, but which are higher than other organic room temperature paint-on semiconductors.
In more detail, the researchers discovered how electrons in their perovskite thin-films interact with other charged particles, and how the soft-flexibility of the non-covalent bonds between the sandwiched inorganic-organic-inorganic lattice contribute to their relatively high performance. Since the material is composed of positively and negatively charged ionic particles, their electrons move within the crystal in coherent vibration modes (phonons) that strongly interact with the ionic lattice. Energetic electrons and holes bind together (as excitons) and move about the lattice periodically orbiting atoms to form polarons. This results in what the researchers call a 'soup' of high-energy interactions that gives rise to complexity that has wider applications.
"We studied these properties with femtosecond laser pulses using stroboscopic methods to determine the interactions that underpin the materials electronic and optical properties," said Silva.
Next, the researchers plan to fabricate a new generation of high-energy electro-optical devices that bond excitons to light particles (photons), resulting in quasi-particles that are part-matter/part-light called polaritons. The polaritons are expected to undergo quantum phase transitions analogous to when a gas condenses into a liquid, but which is stable at room temperature (unlike conventional semiconductors, that must be supercooled to maintain stable polaritons).
"Quantum mechanically, these exotic particles behave like a gas if their density is below a certain threshold, but above that threshold they behave like a quantum liquid made of both light and matter. This liquid exhibits quantum properties like superfluidity [resistanceless motion]," said Silva. "We are interested in exploiting these properties to fabricate devices for quantum optoelectronics applications, such as room-temperature coherent light sources, amplifiers, and perhaps more useful quantum gates."
R. Colin Johnson is a Kyoto Prize Fellow who has worked as a technology journalist for two decades.
Join the Discussion (0)
Become a Member or Sign In to Post a Comment