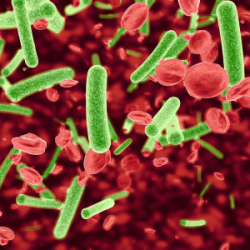
A headache or other pain will send many of us to the medicine cabinet for a pain reliever. Molecules from the swallowed pill quickly find their way directly to the source of the pain. But how do they know where to go? Of course, they don’t; the molecules travel throughout the body, chemically reacting wherever they can.
The consequences of “broadcasting” drugs to the whole body are profound. Drugs that attack rogue, cancer-causing cells also afflict other dividing cells, such as those in the intestine. In fact, chemotherapy doses are often reduced to avoid nausea and other unpleasant side effects, and other, more powerful drugs are too toxic to even be considered.
Researchers have long dreamed of “magic bullets” that go directly where they are needed. Indeed, many current drugs are formulated to be taken up by particular tissues, and nanotechnology is giving researchers even more delivery options. But what if the delivery system could “diagnose” the local conditions? In contrast to today’s “dumb envelopes,” Ehud Shapiro, a computer scientist and biological chemist at the Weizmann Institute of Science in Rehovot, Israel, likens this approach to a “smart envelope.” The envelope “would open up only at the right place and the right time for the specific action,” such as releasing a potent but toxic cancer drug, he says. “This would open up a whole range of molecules that are totally inaccessible today as drugs.”
In addition to delivering drugs, microscopic agents could transform the regeneration of damaged tissues and the diagnosis of disease. The time until these ideas help patients is “probably measured in decades, not in years,” Shapiro admits. Long before that, however, researchers could use the new tools to explore biology in the lab. The challenge of engineering biology, rather than merely observing it, could yield powerful insights into how biological systems work.
Hijacking Biology
Recent years have been revolutionary for biology. The human genome, as well as computer-based tools that measure thousands of biological chemicals simultaneously, have inundated biologists with data about how these chemicals interact to create the processes of life. An eager group of researchers around the world take this data glut as a challenge to build new biological circuits from scratch, in what is known as “synthetic biology.” Using various strategies, they are assembling pieces that might enable completely new approaches to medical technology.
In 2004, for example, Shapiro and his colleagues created a test-tube “molecular computer” consisting of three interconnected modules. The first module sensed the concentrations of four types of messenger RNA, the working copies of the genetic instructions in DNA, which are used to produce proteins. The second module performed a “diagnosis,” computing whether two of the messenger RNA levels decreased while two others increased, a signature that might indicate a disease. Depending on the results of the computation, the third module dispensed a drug molecule. “We demonstrated the whole process, beginning to end,” Shapiro notes, “but in a test tube.”
To both sense specific strands of messenger RNA and to perform the computation, the Weizmann researchers exploited the sequence-specific matching of DNA strands. So far, though, they have not operated their molecular computer in the complex environment of a living cell. Other teams have had success with different schemes. For instance, a group including Shapiro’s former collaborator Yaakov Benenson, now a researcher at the FAS Center for Systems Biology at Harvard University, demonstrated computation—but not sensing—in cultured human kidney cells. They exploited the newly discovered phenomenon of RNA interference, in which the presence of short RNA templates activates cellular mechanisms that suppress protein synthesis for matching messenger RNA.
A Caltech team led by Christina Smolke, now a professor of bioengineering at Stanford University, designed complex RNA molecules that included three separate sections, performing sensing, computation, and actuation. Although all three modules are part of the same molecule, they act independently, so the function of each part can be separately modified, she says. “You have this plug-and-play type capability to build many types of functions from a smaller set of modular components.” The RNA molecules they design are manufactured by yeast or even mammal cells after the researchers insert the corresponding DNA.
In addition to computing Boolean logic operations, Smolke’s team has demonstrated other signal-processing functions, including bandpass filtering and adjustable signal gain, with their RNA platform. But she acknowledges that the field has yet to settle on the best approach. “Ultimately, you want to get to a place where there’s some level of standardization,” she says.
In addition to computing Boolean logic operations, a Caltech team has demonstrated other signal-processing functions with its RNA platform.
Send in the Clones
One barrier to standardization is the wide range of possibilities for using biological agents in medicine. Smolke’s technique, for example, might be used to genetically modify cells in a particular tissue, but she is also exploring modification of immune cells outside of the body to combat cancer. “We’re utilizing the function that [the immune cell] already does really well, and then endowing it with enhanced functions,” she says.
Chris Anderson, a professor in the department of bioengineering at the University of California, Berkeley, envisions a different strategy, one based on engineered bacteria, but admits that “it’s impossible to know what’s going to win out.” A “huge advantage” of using bacteria, Anderson says, is that the biological processes targeted by antibacterial agents are very different from those of human cells, so the engineered bacteria can be easily killed.
For bacteria to be effective, they must be able to evade the body’s immune defenses. Anderson and his colleagues have transplanted genes from other bacteria that allow their E. coli to survive for hours in the bloodstream, instead of just a few minutes. They also introduced growth-control mechanisms into the bacteria, he stressed. “They’re not able to grow without feeding something to the patient.”
In 2006, Anderson and his colleagues unveiled a bacterium they had engineered to invade nearby cells. Importantly, the invasion only occurred under chosen conditions, including lack of oxygen, which often occurs near tumors. Rather than directly combining sensing, computation, and actuation into a single DNA or RNA molecule, Anderson’s genetic modules communicate using smaller molecules, in much the same way as normal cells. When the researchers insert new DNA into the bacteria, they include special sequences that respond to other chemicals in the cell or the environment. They “connect” their modules by inducing this sensitivity to the products of other genes that they insert. In addition, by requiring that two different molecules attach to adjacent regions of DNA, they created the cellular equivalent of an AND gate.
A Need to Communicate
The chemical sensitivity of genes gives cells some ability to communicate with each other. For example, one of the signals that stimulated Anderson’s bacteria to invade was the well-known “quorum-sensing” response that kicks in for some bacteria when they are present in large numbers. Ron Weiss, a professor of electrical engineering and molecular biology at Princeton University, has used the quorum-sensing machinery to build bidirectional communication between two groups of bacteria. The collective behavior constitutes a kind of computation that reflects the interaction between the two strains, each of which could be tuned to detect separate conditions. In some cases, Weiss says, “a cell that specializes in the detection of one condition can do it much better than a cell that tries to do too many things at once.”
From a broader perspective, says computer scientist Tatsuya Suda of the University of California at Irvine, “there’s always communication involved” in micromedicine. The sensing of the environment by the tiny agents is a kind of communication, he notes, as is the dispensing of drugs. As researchers design these tiny communications systems, he stresses, they need to pay careful attention to noise.
In addition to communicating with their environment, microscopic agents may communicate with each other. As an example, Suda cites regenerative medicine, in which the creation of a replacement organ requires coordinated response by many agents. But he admits that, for now, “the state of the art is just trying to find out how they work together as a group, as opposed to how we can take advantage of group behavior.”
For biologically based agents, as for ordinary cells, any communication is likely to occur through the emission and sensing of molecules. In contrast, artificial or hybrid systems incorporating nanometer-scale electronic components might also communicate by ultrasound or radio. In principle, as described by researcher Tad Hogg of Hewlett-Packard Labs, they could signal to point others to medically important locations. In addition, they might be able to transmit information to the outside world.
Augmenting, rather than replacing, the diagnostic strengths of the medical community could be an important early application of micro-medicine, and relaxes the demands for on-board computation and drug delivery. At a minimum, small devices might extend the capabilities of chemicals whose locations are monitored in modern medical equipment. “As those imaging devices advance,” Hogg says, “they should be able to give you some information more than just ‘here’ or ‘not here,’ but what they found” in a particular region, perhaps by combining several important local measurements.
Even before medical applications become practical, Shapiro suggests, the emerging tools could provide new resources for basic biology research. “I think that these types of molecular computing devices might be able to analyze living cells ex vivo and help researchers understand cells without killing them,” Shapiro notes. “These applications are probably measured in years rather than in decades.”
Join the Discussion (0)
Become a Member or Sign In to Post a Comment