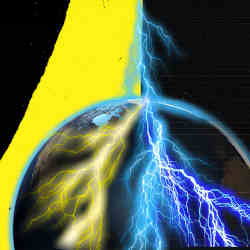
Superconducting qubits, the building blocks of some prototype quantum computers, can be 'poisoned' by ambient radioactivity. The effect of cosmic rays is particularly difficult to shield qubits from. Unless these qubits can be made more resistant to radiation, future quantum computers would need to operate deep underground.
In a practically useful quantum computer, the qubits—the basic computing units of quantum computing—need to stay sufficiently free from outside interference, or the computation will just produce random results. That is why, to minimize thermal noise, quantum processors are cooled to near Absolute Zero.
Research by physicists from the Massachusetts Institute of Technology (MIT) and the U.S. Department of Energy's Pacific Northwest National Laboratory (PPNL) has revealed a source of interference that cannot be frozen out and is very hard to shield off: cosmic rays.
MIT's Antti Vepsäläinen and his team published a paper on this recently in Nature.
For a long time, researchers wondered why superconducting microstructures suffer from a phenomenon called quasiparticle poisoning. Superconduction relies on the free electrons in a metal forming Cooper pairs. While single electrons cannot move through a metal with zero resistance, below a certain critical temperature, pairs of free electrons bond into Cooper pairs which do just that.
Even below the critical temperature, some internal processes were known to break up a certain percentage of the Cooper pairs into quasiparticles (basically, single electrons again), but that fraction was higher than could be explained.
Experiments by the MIT-PPNL group have found that radioactivity (ionizing radiation) coming from the concrete in the laboratory walls and cosmic rays is the culprit for this excess quasiparticle poisoning.
Tim Taminiau, group leader of spin qubit research at QuTech in the Netherlands (and not involved in this research), said, "This is a surprising result, which solves the riddle of quasiparticle poisoning. At least, researchers don't need to worry anymore that this is caused by some flaw in their quantum devices."
In the first experiment, Vepsäläinen and his team put a small radioactive source (64Cu) on top of a chip with two superconducting transmon qubits. This device was put in a cryostat and cooled to near Absolute Zero (-2730 Celsius). By sending microwave signals by wire to the two qubits, they could become 'entangled'—put in a shared quantum state—turning them into the smallest possible quantum computation unit. An important parameter for qubits is their decoherence time, the average time span over which this shared quantum state is lost due to outside interference, after which the qubits need to be reset. By taking the qubits through the cycle of decohering and resetting many times, the decoherence time could be measured accurately.
The radioactive source used in the experiment had a short half-life (13 hours), so the intensity of the radiation decreased greatly over the course of the experiment, which lasted 19 days. The team found a clear, inversely proportional dependence of the decoherence time on the radiation level, demonstrated that, at least at relatively high radiation levels, radiation is the limiting factor for decoherence.
In a second experiment, the cryostat was partly shielded from natural background radioactivity by a cylindrical wall of lead blocks. To measure a statistically significant difference in decoherence time at this much lower level of radioactivity, lead shielding was raised and lowered around the cryostat by a scissor lift many times during the experiment. This corroborated the results from the first experiment.
The team concluded that normal background radiation will limit the decoherence time of this type of qubit to just a few milliseconds.
Said Taminiau, "This is certainly something to has to be taken into account for future quantum computers."
In the past 20 years, the typical time to decoherence of superconducting transmon qubits has increased from nanoseconds to ~0.1 milliseconds, but for a fully operational quantum computer, much longer decoherence times are necessary.
In this laboratory used for the experiments, 60% of the background radiation was coming from the concrete walls (concrete contains traces of uranium and thorium), and the remaining 40% was of cosmic origin. Lead shielding can absorb almost all of the former, but the gamma photons of cosmic rays are extremely penetrating; the only way of completely shielding off cosmic radiation would be to put quantum computers hundreds of meters underground, similar to neutrino detectors.
There are probably ways around that, though. While a quantum computer that outperforms all regular computers would need only a hundred perfect qubits, noise and unavoidable errors will increase that number to thousands of real-world qubits. A hard limit on decoherence time by cosmic radiation of a few milliseconds would make error correction more difficult, and even more qubits would be needed to compensate for that and allow a quantum computer to do useful work. That might be preferable to siting quantum computers deep in abandoned mines.
In addition, while superconducting transmon qubits are used in well-known prototype quantum computers like IBM's Quantum Experience and Google's Sycamore, other types of qubits are much less sensitive to radiation. Taminiau's group at QuTech works with spin qubits in diamond, for which they have already demonstrated 10-qubit processors with a decoherence time of up to one minute.
The simple reason for this huge difference, explains Taminiau, is that "Spin qubits are too small to have interaction with cosmic rays." Although a superconducting transmon qubit looks small, it still consists of billions of silicon and aluminum atoms, which readily absorb radiation. The spin qubit in use by Taminiau's group basically consists of a single nitrogen atom inside a tiny diamond; radiation would have to hit the bull's eye of the single nitrogen atom to decohere the qubit.
While superconducting transmon qubits are still leading the race for application in the first useful quantum computer, quasiparticle poisoning by cosmic radiation may give spin qubits an unexpected boost.
Arnout Jaspers is a freelance science writer based in Leiden, the Netherlands.
Join the Discussion (0)
Become a Member or Sign In to Post a Comment