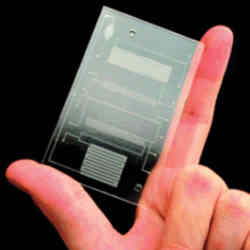
For decades, laboratory procedures have been a popular target for automation; sequencing the human genome, for instance, would not have been feasible without it. Now the scale of automation is being reduced to individual laboratories on a chip by virtue of micron-level manipulation of fluid droplets (microfluidics)—not for complete genome sequencing (yet), but for the myriad of simpler medical procedures today performed by human technicians in full-sized laboratories worldwide.
The main contribution of the lab on a chip, so far, has been the development of medical point-of-care devices that can diagnose specific maladies in minutes, rather than requiring the capture of a blood (or other bodily fluid) sample and its transportation to a lab for analysis.
"Point-of-care diagnostic devices have proved incredibly useful in the last 20 years, in particular delivering much-needed rapid HIV and tuberculosis diagnosis to the developing world [where traditional labs are often not available]," said Maïwenn Kersaudy-Kerh, a professor in Heriot-Watt University's School of Engineering and Physical Sciences and in Scotland's Institute of Biological Chemistry, Biophysics, and Bioengineering, as well as an Honorary Lecturer in the Deanery of Biomedical Sciences of Scotland's University of Edinburgh.
A microfluidic lab on a chip consists of pipe-like micron-sized channels and reservoirs to hold droplet samples—usually blood or other body fluids—to be processed by mixing them with reagents and other chemicals needed to identify a malady. Such a lab on a chip also requires closely integrated electronics to control the processing steps.
A subset of micro-electromechanical systems (MEMS), these microfluidic implementations perform precisely the same steps as in a conventional lab, but using individual droplets of samples instead of test tubes. As a result, they use less reagents and less energy, cost less, and are faster in execution than traditional lab procedures, providing in-house results in minutes, rather than overnight (at best).
Just last month, hepatitis-C diagnoses were added to the list of applications provided by labs on chips. It was demonstrated with a working lab-on-a-chip prototype developed at Florida Atlantic University's (FAU) College of Engineering and Computer Science. According to FAU associate professor Waseem Asghar, about 80% of the 354 million people infected with Hepatitis-C worldwide will develop cancer, cirrhosis, or complete liver failure if not treated. Unfortunately, only about 20% have been identified in developed countries, and only 1% in developing countries.
Asghar said the new "disposable microfluidic lab-on-a-chip device is fully automated and offers reliable diagnoses—by changing a dye color from orange to green—in about 45 minutes and costs only about $2."
Many more medical diagnostic labs on a chip are under development. Many of those are destined not only for point-of-care and in-the-field usage, but for over-the-counter pharmacy shelves as well. This burgeoning development effort has attracted green-minded researchers to analyze the environmental impact of these one-use devices. In hospitals and clinics, for instance, the risk of infection from the body fluids inside require the devices to be incinerated after use. Since many use polymers and other fossil-derived materials, their incineration means a larger carbon footprint for the medical institutions (as burning them releases CO2). If even they are used in the home, the devices will end up in landfills, where cyanide and other toxic chemicals they contain will be released into the environment, according to Kersaudy-Kerh
"The medical waste issue is problematic, with the production of massive amount of plastics, as well as toxic chemical by-products. Now is the time to develop sustainable solutions. Researchers, manufacturers, and practitioners need to come together to design novel solutions using safer, less-polluting, and more easily degradable materials, form-factor reduction and recycling solutions," said Kersaudy-Kerh. "In our lab, we are reviewing existing and possible solutions to make diagnostic development more sustainable, in the hope to inspire and empower researchers to find alternative solutions, as well as reviewing their current practices."
One viable solution is to replace the polymers used in labs on chips with paper-based devices. Off-the-shelf pregnancy tests, for instance, already use paper-based innards within a plastic shell.
Another approach uses paper only, according to New York University Abu Dhabi research scientist Nityanand Kumawat, who explained, "We are engineering a switch to what we call microPADs—microfluidic paper-based analytical devices. These are structurally flexible—virtually impervious to bending, folding, and twisting—as well as exhibiting good chemical resistance to the various reagents and other chemicals used for clinical diagnostics, biological sensing, and food processing. The technique presents a simple two-step process having minimum human intervention, paving the way towards automated mass fabrication of microPADs for a wide range of point-of-care [POC] applications."
According to Kumawat, microPADS are just as low-cost and simple to use as polymer-based labs on chips. They use photoresist-like patterning on porous paper sheets to create fluidic channels through the deposition of wax. His research group is currently demonstrating a simple, inexpensive two-step process—cut and heat—for the fabrication of the necessary microfluidic channels and reservoirs. So far, he said, they have successfully demonstrated 95 proof-of-concept prototypes on biodegradable porous materials including tissue, printing-, and filter-paper, as well as various types of cloth, including cotton.
Another way to boost adoption of lab-on-a-chip technologies, according to Boston University professor of electrical and computer engineering and Biological Design Center member Douglas Densmore, is to simplify the process of designing microfluidic MEMS chips. He and his colleagues recently proposed using machine learning (ML) to acquire the knowledge of expert lab-on-a-chip designers and build it into a software tool that non-experts can use to design new labs on a chip.
"A fully automated computer aided design [CAD] environment would seamlessly integrate machine learning of microfluidics expertise. This would lead to less-expensive, more effective, and more customized designs which would expand the adoption of labs on a chip," said Densmore.
Densmore's research group recently surveyed the state of the art of using machine learning to acquire expertise in the design and control of microfluidic devices, concluding that so far ML is mainly used to simplify the design of individual components. They speculate that by integrating these efforts, a design flow could be created that leads to a "robotic scientist" that non-experts could use to design complete labs on a chip.
Another research group in the U.K., at London's Imperial College and the University of Birmingham, is using deep learning algorithms (recurrent neural networks) to create a high-fidelity data-driven model that predicts how droplets of samples and reagents will interact inside different microfluidic device designs. Their hope is that instead of time-consuming, costly prototyping and tedious measurements of different designs, that the deep learning algorithm can predict the most promising designs before prototyping. Called high-fidelity computational fluid mechanics, the algorithm creates a model that predicts viscosity, surface tension, and interfacial dynamics for droplets' flow fields to predict coalescence probabilities of new designs. Methods of coalescence include active (kinetic) methods, applications of electrical fields, and passive methods using the geometry of coalescence chambers. Their conclusion was that deep learning can create models of microfluidic droplet dynamics that can guide the choices of researchers designing new lab-on-a-chip devices.
Labs on chips also are being researched as alternatives to in vivo experimentation on live humans. As a start, individual organs are already being emulated by microfluidic devices, including the skin, arteries, bones, cartilage, lung, heart, kidney, liver, prostate, and brain. The endgame is to link organs on a chip together into what would be called a human body on a chip (or linked chips), thus mitigating the need for extensive in vivo trials.
R. Colin Johnson is a Kyoto Prize Fellow who has worked as a technology journalist for two decades.
Join the Discussion (0)
Become a Member or Sign In to Post a Comment