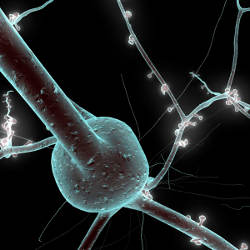
At any given moment, a live mammalian brain is crackling with electrical activity. Neural impulses are zipping along at the speed of a race car, activating millions of a hundred trillion possible connections. The complexity of the brain makes it incredibly difficult to study experimentally. Neuroscience has broken down brain behavior into piecemeal problems, to understand it in bite-sized parts. For one man, even that is not enough.
Henry Markram, chief of the Blue Brain Project, wants to recreate the brain in all its complexity, from the genetic level up to the molecular level to individual cells that form smaller and larger circuits. Run on advanced supercomputers, this digital brain would incorporate several separate individual theories under one unified model.
So far, researchers at the Blue Brain Project have only simulated small sections of brain tissue. But the hope is that with enough complexity fed into it, a future digital brain will exhibit brain-like complex behavior, and serve as a model system to study brain function, cognition, and disease.
The Blue Brain Project is headquartered at the École Polytechnique Fédérale de Lausanne in Switzerland. The project brings together bench work by experimental neuroscientists, code developed by computational neuroscientists, and simulations crafted by visual design experts. Work continues in partner labs in Israel, Spain, and the U.S. to develop separate parts of the project, which will eventually feed into the master simulation.
Now in its seventh year, the Blue Brain Project has made healthy strides toward its goal. In 2008, Blue Brain Project researchers successfully simulated a tiny sliver of a newborn rat brain called a neocortical column—tissue about 0.5 millimeters across and 1.5 millimeters tall—the equivalent of a processor on a laptop. Once the model was in place, they gave the virtual structure a jolt of electricity, and watched neural connections form spontaneously in familiar, natural ways—without having been programmed to connect as they did.
The Blue Brain simulations have yet to demonstrate brain-like behavior, like image recognition or simple memory tasks. Some researchers within the field question whether the path to a model brain that reasons involves reproducing the natural brain in exacting, dense, biological detail. Such skepticism about the role of large-scale brain models was raised anew in November 2012, when a group from the University of Waterloo built a model brain called SPAUN, with two million simple neurons that could perform eight different complex tasks—the kinds of tasks the Blue Brain simulations are far from copying. The SPAUN simulation can “remember” a string of elements in a list, or complete a number sequence by identifying the rule that drove it.
Chris Eliasmith, who has been developing the Waterloo approach for more than 10 years, explains that projects like Markram’s, and his own “SPAUN” approach, share a goal: to understand how the nervous system can be organized to give brain-like behavior, although each uses a different approach. “We got actual function,” Eliasmith says; “it’s actually doing thinking.” Theoretical modelers are skeptical about just adding detail to a model, he explains, and their view is that the brain is collectively more than just a collection of neurons.
Work at the Blue Brain Project’s Swiss HQ carries on. A few months ago, a small group within the BBP described another key result: they created a structurally accurate model of a small section of the brain and, using a Blue Gene/P supercomputer, simulated how connections between the neurons form.
It was a successful virtual model of a biological mechanism, but also an example of how simulations based on years of experimental data can yield insights that are difficult to achieve solely through studying slices of brain tissue.
Location, Location, Location
Neurons vary in shape, but are typically stringy structures with a long shaft—the axon—ending in a cell body. Out of the cell body emerge branching structures called dendrites, making the overall neuron structure look something like a skinny tree. When dendrite meets axon, and information is exchanged—that location is called a synapse. Every neuron has between 1,000 and 10,000 synapses.
The formation of a synapse—this active space of exchange between neuron and neuron—is influenced by the structure and location of neurons. A study published in October in Proceedings of the National Academy of Sciences sought to answer the broad question: How do neurons form meaningful connections between each other, and what does location have to do with it?
“Where the synapses are positioned on the tree makes a difference to where that neuron fires,” says Sean Hill, the primary author on the paper and executive director of the International Neuroinformatics Coordinating Facility. Hill is also a former project coordinator on the Blue Brain Project.
Recognizing where neurons form meaningful connections with other neurons is an essential part of understanding how, at the fundamental level, a neural network forms, regenerates, and works. According to existing theories, neurons follow a trail of chemical breadcrumbs that direct their growth and synapse formation. But where did location come in?
In this reconstruction, researchers focused on the neocortical microcircuit of a two-week-old rat. A neocortical microcircuit is a basic computational unit of the brain, a fundamental circuit at the core of what the brain does. Neocortical circuits process sensory inputs like touch and sound, and the associative functions that link them together. The study has broader implications, because neocortical microcircuits are repeated several times in a single brain, and versions of the circuit with very small differences attend to a range of basic cognitive functions. Among mammals, the neocortical microcircuit is repeated with similar design principles.
In one version of the virtual reconstruction, researchers used 298 different kinds of neurons. Every neuron was unique, placed at the location and level in the column in which it was naturally found. In another version of the neocortical microcircuit column, the team engineered the reconstruction with just one kind of neuron, repeated over and over.
Just by arranging the different kinds of neurons at the right level in the 3D space, in the majority of cases, model synapses occurred at locations in which biological synapses would have formed in a natural system. “We had no expectation that just by throwing on the right neurons at the right levels, that it would match the biological data,” Hill says.
The modeled synapses were compared to what Hill calls an “unprecedented dataset”—biological synapse mapping data that the group collected over more than a decade. The team used whole patch clamp techniques—inserting tiny electrodes into adjacent neurons—to check for communication between neurons. Active synapses were marked in tissue by injecting a small quantity of dye.
This simulation offers one possible indication for why there are so many different kinds of neurons in the brain. When different classes of unique neurons were positioned at specific locations in a neocortical microcircuit column, he explains, the synapses in the model formed at the same statistical location every time. With every single neuron within a class constant, the synaptic arrangement is not as predictable or consistent.
“[The work] is a fundamental step, as it gives construction rules for cortical circuitry that accelerates our building of structurally accurate models,” says Felix Schürmann, general project manager at the Blue Brain Project, and a co-author on the PNAS study.
Alongside the cortical simulation, running in parallel, there’s plenty more research reaching goals on the path to a more complete brain model. Other works, published in PLOS Computational Biology and the Journal of Physiology in the last year, are function studies that bear on the structural details published by Hill and team. Taken together, Schürmann explains, these represent steps that will eventually build up to functional virtual tissue.
This is Your Brain on a Supercomputer
In the meantme, the charismatic leader of the Blue Brain Project, Henry Markram, has already set his eyes on the next big goal. It is called the Human Brain Project, and anticipates the supercomputing power that science will have access to in the next 10 years. The goal of the Human Brain Project is to scale up the unified computer model followed by the Blue Brain Project, but applying it, this time, to the whole human brain. Like the BBP, the HBP will feed into its model everything neuroscience knows about the human brain, and keep it running on a supercomputer. The simulation will include some, but not all, molecular dynamics that influence the brain, like hormonal influences. For example, the model could factor in the input from, say, the thyroid gland, without needing to simulate the thyroid itself.
“We had no expectation that just by throwing on the right neurons at the right levels, that it would match the biological data.”
“[It] won’t contain all the molecular interaction,” Richard Walker, senior science writer at the BBP clarifies. “We hope the level of molecular interaction will be sufficient.” The functioning of the brain would be tested in stages (as the Blue Brain Project currently does). For example, if the model got as far as a model rat brain, it would be asked to navigate a model maze to see how it performed, Walker explains.
Markram estimates that scientists could be running simulations of the whole brain within the next decade using exaflop-scale supercomputers, and has begun rallying support for this grander Human Brain venture. With more than 100 collaborators at 87 institutions waiting at the ready, Markram in late January received approximately
1 billion in funding from the European Commission as part of the Future and Emerging Technologies Flagships Initiatives. European Commissioner for IT Neelie Kroes says the objective of the program, which will provide the funding over a 10-year period, is to “keep Europe competitive, to keep Europe as the home of scientific excellence.”
Markram’s plan is that other scientific groups will borrow time on the whole brain simulator to develop new diagnostic tests for diseases, or test new theories about how diseases progress and develop. “[The HBP] will furnish a framework for what we know, while enabling us to make predictions of what we do not,” Markram wrote in his introduction to the Human Brain Project published in Scientific American in June 2012. “Those predictions will show us where to target our future experiments to prevent wasted effort.”
In the coming decade, Markram anticipates advances in supercomputing that will make the Human Brain Project possible, as well as limitations to that same computational power needed to run such a brain. He believes the Human Brain Project itself will suggest new insights for how to build supercomputers based on the brain’s natural design, giving back to the same field that made it possible in the first place.
Further Reading
Eliasmith, C., Stewart, T. C., Choo, X., Bekolay, T., DeWolf, T., Tang, Y., Rasmussen, D.
A large-scale modeling of the functioning brain. Science 338, 12021205, 20, Nov. 2012.
Hill, S. L., Wang, Y., Riachi, I., Schürman, F., Markram, H.
Statistical connectivity provides a sufficient foundation for specific functional connectivity in neocortical neural microcircuits, Proceedings of the National Academy of Sciences, 18, Sept, 2012.
Markram, H.
The Blue Brain Project, Nature Reviews Neuroscience, 7, Feb 2006.
Markram, H., and Segev, I.
Augmenting Cognition, EPFL Press, Lausanne, Switzerland, 2011.
TEDGlobal
Henry Markram at TED 2009: Supercomputing the brain’s secrets, http://www.ted.com/talks/henry_markram_supercomputing_the_brain_s_secrets.html, July 2009.
Join the Discussion (0)
Become a Member or Sign In to Post a Comment